Wearable sensors are portable, low-cost, and unobtrusive devices that have the potential to alter rehabilitation in the clinical setting. This potential can be realized through monitoring the health condition of an individual as they perform daily activities
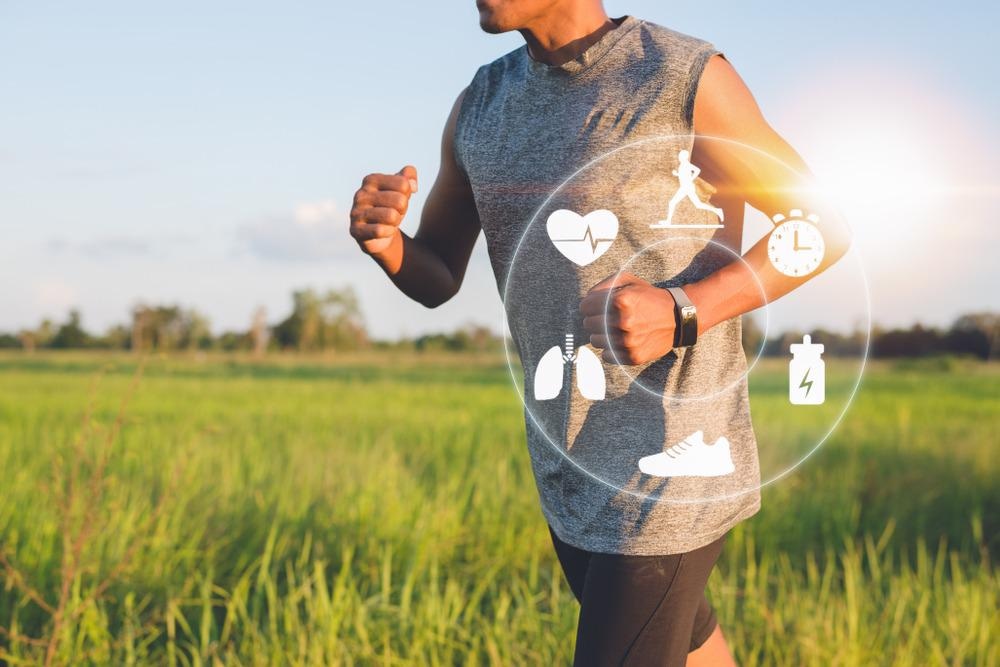
Image CreditL sutadimages/Shutterstock.com
What Are Wearable Sensors?
Wearable sensors have monitoring as well as diagnostic capabilities. Monitoring capabilities encompass physiological and biochemical, and motion sensing. Over the past decade, these sensors' focus has predominantly concentrated in the healthcare industry, where these noninvasive and real-time wearable sensors allow for continuous individual surveillance.
The supply of adequate information necessary to elucidate health status – including preliminary medical diagnosis, can be made easier through wearable sensor technology.
Wearable biosensors take the form of clothing, glasses, watches, and bandages that are conveniently attached to a person's body. Evolutions in technology have facilitated their expansion to include accessories and body insertions, including implantable and ingestible devices that enable diagnosis and prognosis.
The remote and long-term patient monitoring capability necessary for rehabilitation is enabled by their connectivity. Wearable devices are interoperable as they connect to a range of intelligent devices via infrared, radio-frequency identification (RFID), Bluetooth, and near-field communication (NFC).
Harnessing Wearable Sensor Technology for Rehabilitation
Wearable sensors have been translated into the field of rehabilitation. The aim of rehabilitation is the recovery of motor function, particularly in the fields of neurology and orthopedics. Rehabilitation interventions facilitate motor learning by harnessing task-specific tasks that are repetitive and progressive in design to enhance activity-dependent plasticity in the central nervous system.
Rehabilitation is dependent on the ability to monitor the human body and its dynamics to support tailoring support exercise and chemical and physical interventions. In this context, bioelectric sensors, added to inertial sensors and pressure sensors, are implemented to control movements and evaluate the function of muscles. In addition to these sensors, stimulus systems such as virtual reality are used as auxiliary platforms for rehabilitation.
Examples of Wearable Sensor Use in Rehabilitation
Orthopedic and neurological issues comprise a majority of rehabilitative patients – particularly among the elderly. Force-based sensors are the most popular form utilized in wearable sensors for rehabilitation. Several forms of these sensors are used to provide auditory, visual, and biofeedback alongside force-based measurements. These force-based sensors include gyroscopes, accelerometers, and magnetometers.
These types of sensors are combined with inertial measurement units (IMUs), which optimize these force-based sensors.
Falls management is used in identifying differences in kinematic gait variables in post-stroke patients and identifying differences in gait stability between stroke and control populations, as well as investigating variables that may play a significant role in increased fall risk
Defining pathological motor features can form part of rehabilitation as bradykinesia (slow movement), hypokinesia (decreased amplitude or range of movement), and dysrhythmia (irregular heartbeat rhythm); for example, for those with Parkinson's disease can be monitored and used to inform changes which can be fed into physical therapy plans
Recognizing activity is the ability to classify basic activities common to daily life in individuals who have suffered a stroke and monitor activity levels, assess real-world performance, and guide community-based treatments for individuals who have suffered a stroke without the need for relying on self-reported data.
Biofeedback is used in home-based rehabilitation in individuals with Parkinson's disease, and those who have suffered a stroke as biofeedback can be used to improve balance, range of motion, strength, and mobility comparable to therapist guided rehabilitation
A Taste of The Future: A Recent Example of Wearable Sensors in Rehabilitation
The most recent example of wearable biosensors for rehabilitation is a nanomaterial-based sensor that can determine musculus fatigue from sweat. This device is composed of an ultra-thin nanomaterial known as MXene. MXenes are a class of 2D materials comprised of non-toxic metals in combination with carbon or nitrogen, affording them the characteristics of high conductivity and strong surface charges.
By enclosing an MXene composite electrode in a wearable armband, researchers designed a modular system, loaded with appropriate enzymes, to absorb perspiration and analyze several analytes in human sweat, including glucose and lactic acid.
This prototype follows muscular movement; mechanical stress produces distinct electrical resistance patterns that are further influenced by the presence of ions in the form of acidic or basic solutions—increased mechanical load results in increased muscular fatigue, which produces lactic acid. This is translated as increased pH of sweat.
Therefore, the sensor is capable of tracking muscular movements and changes in pH of sweat; translating this technology enables those suffering from muscular damage to avoid overexerting themselves during rehabilitation.
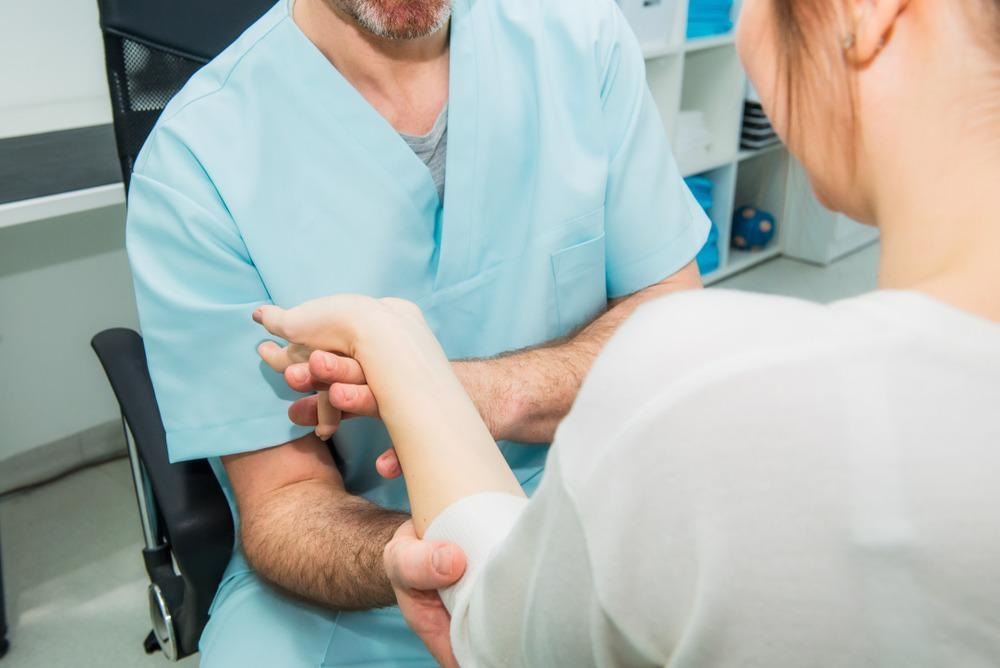
Image Credit: Okrasiuk/Shutterstock.com
Current State, Limitations and Future Direction of Wearable Sensor for Rehabilitative Purposes
The industry is experiencing a growth phase, with much of its potential to be realized as advancements in materials science allow for the development of next-generation sensors. At present, wearable sensor use is limited.
Major barriers to wearable sensor technology originate from current clinical practice and the limitations of the wearable sensors themselves. The barriers posed by the clinical practice include a busy clinical environment and an underappreciation of the value that wearable senses bring.
One limitation is the poor accuracy of consumer-grade devices produced for physical rehabilitation patient populations i.e., susceptibility to mechanical wear over time, limited direct measurements, sensitivity to temperature, pH and humidity.
Another common issue is that research-grade devices tend not to be user-friendly and require extensive training. A lack of published data on the reliability, validity, and responsiveness of the device’s output also prevents informed clinical decisions from taking place.
Although the introduction of flexible materials enables sensor miniaturization, significant advancements in power supply, data transmission and biofouling of materials must be addressed.
Looking to the future, it is expected that wearable sensors will become more agile, accurate, robust, and reliable and be more readily incorporated into fashion accessories that are already part of the daily lives of their uses. With this, wearable sensors will become increasingly less invasive, helping to democratize healthcare as users can be monitored remotely and in the comfort of their homes.
Continue reading: Wearable E-Nose Sensor Detects Liver Dysfunction.
References and Further Reading
Liu X, Zhao C, Zheng B, et al. (2021) Wearable Devices for Gait Analysis in Intelligent Healthcare. Front Comput Sci. 2021;3:661676. https://www.frontiersin.org/articles/10.3389/fcomp.2021.661676/full
Maceira-Elvira P, Popa T, Schmid AC, Hummel FC. (2019) Wearable technology in stroke rehabilitation: towards improved diagnosis and treatment of upper-limb motor impairment. J Neuroeng Rehabil. 2019;16(1):142. https://jneuroengrehab.biomedcentral.com/articles/10.1186/s12984-019-0612-y
Boukhennoufa I, Zhai X, Utti V, et al. (2022) Wearable sensors and machine learning in post-stroke rehabilitation assessment: A systematic review. Biomedical Signal Processing and Control. 2022;71. https://www.sciencedirect.com/science/article/pii/S1746809421007941
Porciuncula F, Roto AV, Kumar D, et al. (2018) Wearable Movement Sensors for Rehabilitation: A Focused Review of Technological and Clinical Advances [published correction appears in PM R. 2018;10(12):1437]. PM R. 2018;10(9 Suppl 2):S220-S232. https://onlinelibrary.wiley.com/doi/10.1016/j.pmrj.2018.06.013
Lee KH, Zhang YZ, Kim H, et al. (2021) Muscle Fatigue Sensor Based on Ti3 C2 Tx MXene Hydrogel. Small Methods. 2021;5(12):e2100819. https://onlinelibrary.wiley.com/doi/abs/10.1002/smtd.202100819
Disclaimer: The views expressed here are those of the author expressed in their private capacity and do not necessarily represent the views of AZoM.com Limited T/A AZoNetwork the owner and operator of this website. This disclaimer forms part of the Terms and conditions of use of this website.