In this article, flexible sensors are introduced, describing how they work and their applications to industries like biomedical science.
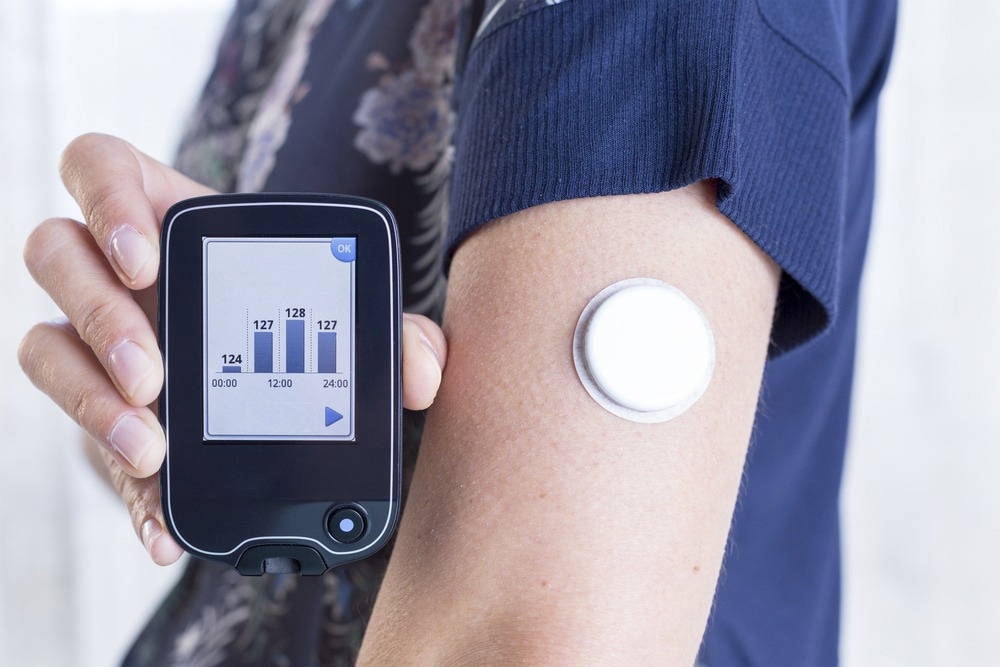
Image Credit: Click and Photo/Shutterstock.com
What is a Flexible Sensor?
Flexible sensors are, broadly speaking, any sensing device able to withstand mechanical deformation, such as bending, folding, and twisting. This imparts a variety of additional functionalities to sensors in a wide range of fields, as they are better able to detect dynamic movement than typical small rigid sensors and can incorporate a number of electrical components into a single system.
Flexible sensors can be constructed from a variety of materials, at their most basic, from flexible plastics with imbued electronic components. Molecular probes and sensors are frequently incorporated into modern, flexible sensors for the highly specific detection of low-concentration analytes. Here, small molecules or biomolecules with a complementary structure to the target are bound to the flexible sensor.
A nearby fluorophore or colorimetric indicator may be activated upon bonding, such as the induced agglomeration of gold nanoparticles, causing a shift in surface plasmon resonance wavelength and, thus, visual color change. Similarly, small molecule compounds, metal nanoparticles, and fluorescent biomolecules can be utilized as indicators of changes in other physical and chemical conditions, such as a change in temperature or local pH.
How Does a Flexible Sensor Work?
Depending on their intended application, flexible sensors may utilize a wide range of sensing technologies. Disposable or re-usable colorimetric or fluorimetric sensors that are able to indicate the presence of a particular analyte may contain a fluorophore and quenching agent that separate or come together in the presence of the analyte owing to structural changes caused by bonding, or metallic nanoparticles exhibiting surface plasmon resonance may be brought together or separated, inducing a shift in surface plasmon resonance frequency.
Flexible sensors of physical movement and pressure are constructed from flexible and biocompatible layered polymers containing metallic or carbon nanowires, where movement is inferred by the degree of contact between wires and the change in the local resistivity. For example, a simple pressure sensor may consist of corrugated gold nanowires wedged between two flat electrode layers, such that when the layers are compressed, a greater degree of contact is achieved between electrodes, indicative of the degree of pressure.
Many flexible sensors utilize cyclic voltammetry to detect the presence of analytes or other physical changes. Cyclic voltammetry is a type of potentiodynamic measurement wherein the potential difference between electrodes is ramped up and down, and the resulting current at the working electrode is measured.
The presence of analytes absorbed onto the electrode, or other changes to the local chemical environment, produce a differing current in response to the changing potential difference that can be interpreted qualitatively. The abovementioned conductive nanomaterials and small molecule or nanomaterial probes can be utilized within a cyclic voltammetry set-up, allowing the detection of picomolar quantities of analyte or other minor changes in the probe environment.
Several methods may power flexible sensors but generally incorporate a continuously self-generating micro-power supply rather than batteries. For example, the piezoelectric effect and capacitance can be exploited in sensors expected to move, wherein piezoelectric nanomaterials generate an electric charge in response to applied stress.
Triboelectric phenomena have also been exploited to power flexible sensors, as observed when charging a balloon with static electricity by rubbing on a material with higher electrochemical potential. In this case, a positively charged layer, the “charge collecting layer” is separated from a negatively charged layer, the “charge generating layer”, by a “charge trapping layer”.
When the charge-collecting layer is touched by skin the charge between the sensor and skin is equalized. Upon separation, a net positive charge is imparted to the charge-collecting layer. Current flow is then achieved as the potential difference between the charge collecting and charge trapping layers equalizes, while further energy is provided by subsequent incidental touching of the flexible sensor during ordinary use.
Data storage hardware is not usually incorporated into flexible sensors, in favor of wireless technologies such as Bluetooth and IoT
Where are Flexible Sensors Used?
Flexible sensors have numerous applications in a wide variety of fields; in any function that may benefit from non-traditional sensor hardware by imparting flexibility in a low-profile and adaptable platform. For example, flexible sensors are set to bring about a revolution in food packaging, where they can be imbued directly into packaging to sense changes in temperature, pH, air exposure, and the presence of pathogens.
Perhaps the most driven ultimate application for flexible sensors is wearable biomedical sensors, whose flexibility and range of biocompatible construction materials allow them to be worn continuously without impediment. For example, flexible sensors for glucose can be worn by diabetic patients, and their blood glucose level is continuously transmitted to an external device or phone application.
Any number of biomarkers of disease and disease progression can be monitored in this manner using flexible sensors, and similarly, the concentration of therapeutic drugs in circulation is monitored where sensitive administration is required. Flexible sensors specifically intended to monitor various other physiological parameters are also under development, such as motion sensors monitoring the progression of Parkinson’s, and general sensors of physiological state intended for use by emergency services and soldiers.
Flexible Sensors: Commercial Landscape
While flexible sensors intended for use in the biomedical field can be produced from biocompatible materials, these materials are typically easily degraded in high-wear situations. The development of high-stability biocompatible materials is, therefore, a priority in the development of flexible sensors. Developments in wireless power transfer and the further miniaturization of energy storage devices will allow flexible sensors greater functionality in the future, and combined with durable, high-stability materials, will allow their widespread commercialization.
In recent years the substrate component has been done away with in some cases by directly adhering the sensing components to the skin, known as “smart tattoos”. Nanowires and triboelectric or piezoelectric nanogenerators can be attached directly to the skin using a biocompatible adhesive. Furthermore, the volume of internally implanted devices, both traditional rigid or flexible sensors, can be spared by wireless communication with temporarily applied external flexible sensors, fulfilling charging and data transfer roles.
References and Further Reading
Costa, J. C., Spina, F., Lugoda, P., Garcia-Garcia, L., Roggen, D., & Münzenrieder, N.. (2019). Flexible Sensors—From Materials to Applications. Technologies, 7(2), p. 35. https://www.mdpi.com/2227-7080/7/2/35
Zhao, H. et al. (2016) Microchip based electrochemical-piezoelectric integrated multi-mode sensing system for continuous glucose monitoring. Sensors and Actuators B: Chemical, 223, pp. 83-88. https://www.sciencedirect.com/science/article/abs/pii/S0925400515303294
Lei, H. et al. (2021) Advances in self-powered triboelectric pressure sensors. Journal of Materials Chemistry A, 9, pp. 20100-20130https://pubs.rsc.org/en/content/articlelanding/2021/ta/d1ta03505c
Zazoum, B., Batoo, K. M., & Khan, M. A. A.. (2022). Recent Advances in Flexible Sensors and Their Applications. Sensors, 22(12), p. 4653. https://www.mdpi.com/1424-8220/22/12/4653
Zhang, Y., Yang, J., Hou, X., Li, G., Wang, L., Bai, N., Cai, M., Zhao, L., Wang, Y., Zhang, J., Chen, K., Wu, X., Yang, C., Dai, Y., Zhang, Z., & Guo, C. F.. (2022). Highly stable flexible pressure sensors with a quasi-homogeneous composition and interlinked interfaces. Nature Communications, 13(1), p. 1317 https://www.nature.com/articles/s41467-022-29093-y
Luo, Y., Abidian, M. R., Ahn, J.-H., Akinwande, D., Andrews, A. M., Antonietti, M., Bao, Z., Berggren, M., Berkey, C. A., Bettinger, C. J., Chen, J., Chen, P., Cheng, W., Cheng, X., Choi, S.-J., Chortos, A., Dagdeviren, C., Dauskardt, R. H., Di, C.-A., … Chen, X.. (2023). Technology Roadmap for Flexible Sensors. ACS Nano, 17(6), pp. 5211–5295. https://pubs.acs.org/doi/10.1021/acsnano.2c12606
Disclaimer: The views expressed here are those of the author expressed in their private capacity and do not necessarily represent the views of AZoM.com Limited T/A AZoNetwork the owner and operator of this website. This disclaimer forms part of the Terms and conditions of use of this website.