Diabetes is a disease wherein the pancreas no longer produces the insulin required by the body, or alternatively, the body cannot utilize the produced insulin, causing dysregulation of blood glucose levels. Mild symptoms of diabetes range from thirst and increased urinary frequency to much more severe complications, including kidney disease, cardiovascular disease, long-term disability, and death.
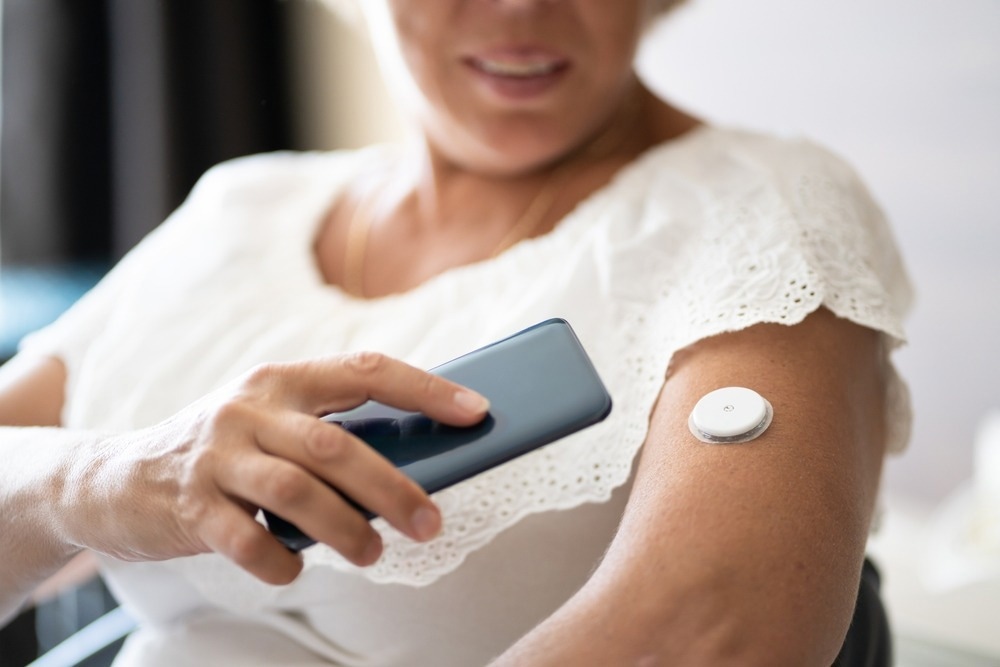
Image Credit: Andrey_Popov/Shutterstock.com
Monitoring of blood glucose levels is therefore of extreme importance for diabetic patients and is typically achieved by the lancet collection of a small quantity of blood from the finger to test blood glucose levels. However, this collection form can be painful and inconvenient and does not inform of blood glucose spikes outside of testing times.
Microelectronic systems are increasingly capable of continuous blood glucose monitoring in a manner feasible for ordinary users during day-to-day life.
How Do Blood Glucose Sensors Work?
Most blood glucose sensors operate by electrochemical principles and utilizing enzymes, either measuring oxygen consumption or hydrogen peroxide production during glucose oxidase enzyme-facilitated reactions between glucose and flavin adenine dinucleotide, or by measurement of electron transfer from glucose oxidase enzymes to an electrode.
Thus, higher reaction product concentration or electrical current is indicative of higher glucose concentration and higher blood sugar level. Continuous microelectronic blood sugar monitors have been in development since the 1970s and 80s, with the first in vivo example demonstrated in 1982. Subcutaneous blood sugar monitors currently in existence typically operate in the same manner as modern prick test glucose monitors and utilize fine needles to control the flow of interstitial fluid into the device; due to concerns surrounding contamination and coagulation risks, monitors do not contact blood directly.
Newer innovations utilizing dialysis membranes have further separated the interstitial fluid from the transducer, and offer greater precision and accuracy than needle-type sensors.
A modern continuous blood glucose monitor developed by Chien et al. (2022) utilizes percutaneous microneedle arrays constructed from 1 mm long, 0.1 mm thick stainless steel needles. One half of the needle array is coated with gold to act as a counter electrode, while the other has polyaniline and gold as the working electrode, with a section coated in silver chloride as a reference electrode.
The glucose oxidase enzyme is then entrapped within the porous polyaniline layer, which acts as a conductive polymer to transfer electrons. An additional 2-hydroxyethyl methacrylate (HEMA) layer was then placed over the needles to protect the enzyme from degradation and act as a glucose-limiting membrane.
Within the circuit, glucose and oxygen react with the act of glucose oxidase to produce gluconolactone and hydrogen peroxide; the working and counter electrode have currently passed through them while the reference electrode remains neutral.
During the chemical reaction, electrons can be transferred to the working electrode and thus change the current there, quantitatively indicative of the ongoing generation and degradation of hydrogen peroxide. Only small voltages around 5 V and extremely small currents of less than 100 nA are utilized by the device, meaning that very small batteries can be used and the device is constructed on a chip around the size of an SD card. The microneedle array is held in place on the arm using a band or tape, and causes no bleeding or notable wounding upon removal.
What Types of Noninvasive Blood Glucose Monitors Have Been Made?
Several types of noninvasive blood glucose monitors have also been developed, largely utilizing optical methods of analysis to detect glucose transdermally or in the optical chamber of the eye. For example, infrared spectrometers have been incorporated into wearable wristbands that are capable of penetrating several millimeters into the skin in the detection of glucose.
Unlike Fourier-transform infrared spectrometers intended for the analysis of a wide range of compounds, and thus must excite the sample through a wide range of infrared wavelengths to detect atomic bonds in resonance, blood glucose monitors can be set to specifically emit light at the wavelengths most relevant to glucose. However, many types of infra-red are relatively poorly penetrating through human tissue, largely absorbed by the large concentration of water in the human body.
Other analytical techniques, such as Raman spectroscopy, have also been used in noninvasive glucose monitors, which function by detecting light scattering off of glucose molecules in association with vibrational modes of the composite atomic bonds and is less affected by water and other environmental changes than infra-red spectroscopy, though as low power lasers must be used against the human body, the technique can suffer from a low signal to noise ratio.
Techniques based on occlusion spectroscopy, optical polarimetry, photoacoustic spectroscopy, microwave spectroscopy, and other analytical technologies have also been adapted to the purpose of blood glucose monitoring. Still, most are difficult to miniaturize beyond handheld systems at this stage, though they could prove useful as alternatives to the invasive prick test.
Conclusion
In recent decades, the number of diabetes patients has increased dramatically on a global scale, generally with the increased availability of high-sugar foods that constitute a large portion of Western diets. Although not implicitly fatal, diabetes can lead to serious complications and even death, and generates a significant healthcare burden regarding ongoing medical evaluation and treatment.
By better managing blood glucose levels on a continuous basis, diabetic patients can significantly reduce the chances of future negative outcomes. In severe cases of blood glucose dysregulation, where constant blood glucose monitoring and correction are required, automated insulin pumps may be utilized that directly provide insulin into the fatty tissue under the skin via a cannula and microfluidic device, and in future, such devices may be integrated as standard into blood glucose monitors for the average diabetic patient.
References and Further Reading:
Chien, M.-N., et al. (2022). Continuous Glucose Monitoring System Based on Percutaneous Microneedle Array. Micromachines, 13(3), p. 478. doi.org/10.3390/mi13030478
Hina, A., & Saadeh, W. (2022). Noninvasive Blood Glucose Monitoring Systems Using Near-Infrared Technology—A Review. Sensors, 22(13), p. 4855. doi.org/10.3390/s22134855
Yen, C.-T., et al. (2022). Noninvasive Blood Glucose Estimation System Based on a Neural Network with Dual-Wavelength Photoplethysmography and Bioelectrical Impedance Measuring. Sensors, 22(12), p. 4452. doi.org/10.3390/s22124452
Yoo, E.-H., & Lee, S.-Y. (2010). Glucose Biosensors: An Overview of Use in Clinical Practice. Sensors, 10(5), pp. 4558–4576. doi.org/10.3390/s100504558
Disclaimer: The views expressed here are those of the author expressed in their private capacity and do not necessarily represent the views of AZoM.com Limited T/A AZoNetwork the owner and operator of this website. This disclaimer forms part of the Terms and conditions of use of this website.