Continue reading to learn more about the challenges associated with developing quantum sensors in this AZoSensors article.
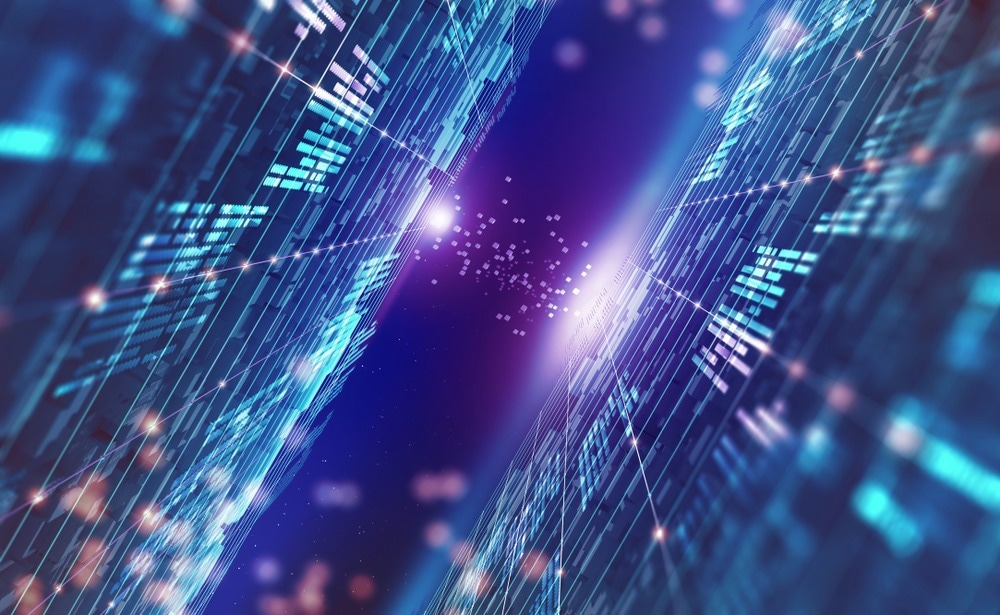
Image Credit: Yurchanka Siarhei/Shutterstock.com
Introduction to Quantum Sensors
Quantum sensors are devices that utilize principles from quantum mechanics to make highly precise measurements of physical quantities, surpassing the capabilities of classical sensors in specific applications by leveraging quantum properties such as superposition and entanglement to achieve enhanced sensitivity and accuracy.1,2
Quantum sensing can be described as using quantum objects like trapped ions, neutral atoms, and spin qubits, using quantum coherence or temporal superposition states to measure physical quantities. It can also be described as improving the precision or sensitivity of a measurement beyond traditional sensing.
Quantum sensors adhere to four key attributes akin to DiVincenzo criteria for quantum computation. These include discrete energy levels, the ability to initialize and readout states, and coherent manipulation through time-dependent fields, enabling precise measurements in quantum systems.3
There are several types of quantum sensors used in quantum sensing applications. For instance, a Superconducting Quantum Interference Device (SQUID) is one of the earliest quantum sensors to measure magnetic fields with 10 aT Hz−1/2 sensitivities.1 These sensors are employed in various fields, including physics, metrology, navigation, medical imaging, and environmental monitoring.
Importance of Quantum Sensors in Advanced Technologies
In advanced technologies such as quantum computing, quantum communication, and medical imaging, quantum sensors perform better than traditional sensors by measuring physical quantities, such as magnetic fields, gravitational forces, and electric fields, with better precision. Similarly, quantum sensors have the potential to enhance the sensitivity and resolution of devices like magnetic resonance imaging (MRI) machines and magnetic spectroscopies. For instance, researchers introduced a groundbreaking quantum sensing protocol that surpasses traditional limits in frequency precision in a recent study.7
The researchers demonstrated nanoscale magnetic spectroscopy with sub-millihertz resolution by combining a single spin sensor in a diamond with a classical clock. Unlike conventional techniques that rely on the coherence time of quantum sensors, this protocol, termed Quantum Heterodyne (Qdyne), utilizes a stable classical clock, enabling a frequency resolution beyond the quantum sensor's coherence time. The researchers successfully applied Qdyne to achieve an 8-order-of-magnitude improvement in spectral resolution over existing methods, showcasing its potential for high-resolution magnetic spectroscopy and nanoscale nuclear magnetic resonance (NMR) applications.7
Technical Challenges in Quantum Sensor Development
Quantum sensing has several limitations that hinder its full potential. For instance, noise and decoherence are significant challenges since, due to the extremely sensitive nature of quantum sensors, the environmental disturbance causes quantum sensors to lose coherence, leading to measurement errors.
Another challenge is scalability since most sensors have small numbers of qubits, which limit their large-scale development. However, some solutions can mitigate these limitations, such as noise and decoherence impact, which can be reduced using quantum controls, dynamic decoupling, and error correction codes. Similarly, researchers have been developing novel materials that could potentially solve scalability issues as well.4
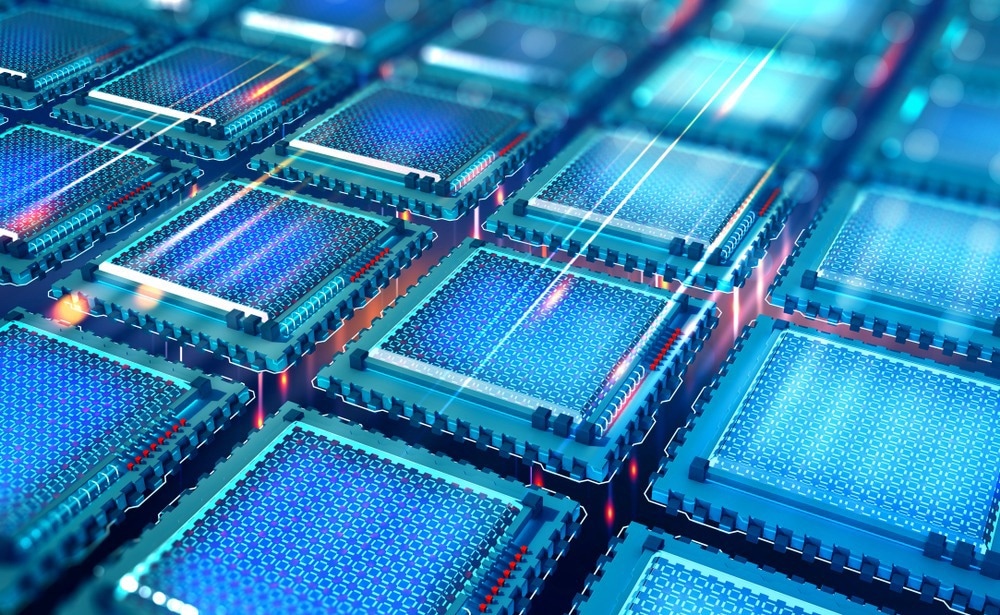
Image Credit: Yurchanka Siarhei/Shutterstock.com
Overcoming Hurdles in Quantum Sensor Development: Critical Areas
It is crucial to explore new materials and engineering techniques to create robust and scalable quantum systems with longer coherence times; hence, diamond, silicon carbide, and various superconducting materials are actively investigated for their potential in quantum sensor applications.
Researchers are putting efforts into reducing the challenges associated with noise. For instance, in a recent study, researchers successfully demonstrated the quantum characteristics of an atomic spin oscillator operating at frequencies below 1 kHz. They mainly focused on observing three main quantum phenomena: ponderomotive squeezing (a method to generate squeezed states of light), induced disturbance due to quantum spin measurement or quantum back-action (QBA), and virtual spring softening in the acoustic frequency spectrum.5
The study addresses quantum noise sources inherent in spin oscillators operating in the near-DC frequency range, which are crucial for various applications, including gravitational wave detectors. Researchers identified these noise sources and presented means for their mitigation, marking a significant step towards quantum noise reduction and enhanced sensing of acoustic frequency signals. The researchers tackled the challenge of noise in quantum sensing, offering insights into the manipulation of ponderomotive squeezing and virtual oscillator-frequency downshift for improved performance in low-frequency acoustic sensing.5
Future Prospects and Potential Solutions
The field of quantum sensor development is rapidly evolving, with significant progress being made on various fronts. The most important factor for the future of quantum sensing is research and development, which is not possible without significant investments in quantum technologies. The trend of investment in quantum technologies is growing, as mentioned by a 2019 news article titled "Quantum Gold Rush", which suggests that 53 quantum technology companies were funded by private investors between the years 2012 and 2019, with a noteworthy increment of funding from 2017 to 2018. 6
Similarly, the development of novel materials with tailored properties that show improved quantum sensing is imperative. Quantum sensors' miniaturization and seamless integration with classical electronics will also be crucial for real-world applications.
Conclusion
Despite technical hurdles like noise and scalability, quantum sensors offer superior precision for advanced technologies. While overcoming these challenges requires research, novel materials, and miniaturization, ongoing advancements and increasing investment will allow a promising future for quantum sensing to revolutionize fields like healthcare, communication, and physics.
References and Further Reading
- Aslam, N., Zhou, H., Urbach, E. K., Turner, M. J., Walsworth, R. L., Lukin, M. D., & Park, H. (2023). Quantum sensors for biomedical applications. Nature Reviews Physics. https://doi.org/10.1038/s42254-023-00558-3
- Jiang, Z., Cai, H., Cernansky, R., Liu, X., & Gao, W. (2023). Quantum sensing of radio-frequency signal with NV centers in SiC. Science Advances. https://doi.org/10.1126/sciadv.adg2080
- Degen, C. L., Reinhard, F., & Cappellaro, P. (2017). Quantum sensing. Reviews of modern physics. http://dx.doi.org/10.1103/RevModPhys.89.035002
- Challenges and Limitations of Quantum. Retrieved on February 22, 2024 from https://fastercapital.com/startup-topic/Challenges-and-Limitations-of-Quantum.html
- Jia, J., Novikov, V., Brasil, T. B., Zeuthen, E., Müller, J. H., & Polzik, E. S. (2023). Acoustic frequency atomic spin oscillator in the quantum regime. Nature Communications. https://doi.org/10.1038/s41467-023-42059-y
- Khan, Taha. (2024, January 29). Where is Quantum Technology Receiving the Most Investment?. AZoQuantum. Retrieved on February 22, 2024 from https://www.azoquantum.com/Article.aspx?ArticleID=491
- Schmitt, S., Gefen, T., Stürner, F. M., Unden, T., Wolff, G., Müller, C., ... & Jelezko, F. (2017). Sub-millihertz magnetic spectroscopy with a nanoscale quantum sensor. https://doi.org/10.1126/science.aam7009%3B
Disclaimer: The views expressed here are those of the author expressed in their private capacity and do not necessarily represent the views of AZoM.com Limited T/A AZoNetwork the owner and operator of this website. This disclaimer forms part of the Terms and conditions of use of this website.