ASHRAE 15-2016 requires that each machinery room should have a refrigerant monitor that can identify a leak at a value not greater than the corresponding threshold limit value.
This would require accurate detection of less than 50 ppm for many of the most frequently used refrigerants such as R123. ASHRAE 147 recommends a stricter requirement on large refrigerating systems that use chlorine-containing refrigerants.
HCFC’s, CFC’s and HFC’s can contribute to global warming and CFC’s and HCFC’s can contribute to depletion of the stratospheric ozone layer. This means a refrigerant monitor which can detect refrigerant concentrations of 1ppm or lower needs to be used on these systems to give early warning of leaks.
Newest Method of Gas Detection
Photoacoustic infrared technology is the newest method of gas detection. It enables gases to be detected at extremely low levels because of its inherent stability and reduced cross-sensitivity. It is critical to choose a gas detector that gives the most accurate and reliable monitoring possible when safeguarding your employees from toxic refrigerant gases. Using the best technology available is your best — and sometimes only — choice.
Infrared Technology in General
It is important to understand how traditional infrared technology works, to then understand how photoacoustic infrared technology works. Infrared detection applies infrared light to detect the existence of gas. A gas absorbs some of the light’s energy when it is exposed to the infrared light.
Specific gases absorb light at certain wavelengths, allowing gases to be identified by gauging the absorption of light at these wavelengths. Optical filters are utilized to pass only the particular band of wavelengths for the gas of interest.
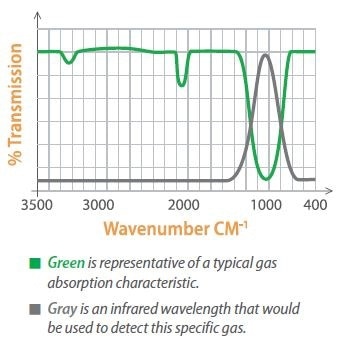
Absorptive Infrared Technology
Historically, one of the most routinely used types of infrared detection has been absorptive infrared technology. In an absorptive infrared monitor, a specific gas sample is exposed to infrared light after being introduced into the measurement chamber of the monitor.
At the same time a sample of an inert gas (typically nitrogen) is kept in a different measurement chamber contained in the same monitor, this is known as the reference gas. One ensures that no absorption takes place and that all the infrared light passes through the chamber by using an inert gas. This gives a baseline from which to calculate light absorption of the selected gas.
The detector makes a comparison between the amount of light transmitted through the reference and the sample cells. The monitor can then establish the concentration of gas that exists in the sample by the ratio of light that is transmitted by the sample gas to the light that is transmitted by the reference gas.
The sample cell does not contain the gas of interest if the amount of light transmitted through both cells is the same. So, the difference between the amount of light transmitted through the sample and reference cells can be used to quantitatively confirm the concentration of gas in the sample cell.
Zero stability for absorptive type instruments are a concern due to contamination, normal aging and ambient effects:
1. Contamination
Any residue due to solvents, water or dust that enters the sample cell can block light between the detector and the IR source. Consequently, the outcome will be an imbalance between reference cell signal and sample. This imbalance can compromise the monitor’s ability to accurately detect refrigerant gas and cause false alarms.
2. Normal Aging
Rezeroing of the refrigerant monitor is required to retain the balance between the sample and the reference cell. This is because, the zero of the absorptive IR unit can drift due to fluctuations in detector sensitivity, IR source light intensity, and/or contamination of the sample cell. The unit could become desensitized to the gas of interest if the sensor degrades without rezeroing.
3. Ambient Effects
Changes in temperature and pressure can affect zero readings (no refrigerant present) on absorptive units, meaning a positive or negative shift in the zero reading can happen. Auto-zeroing can need maintenance intensive filtration or complex zeroing schemes. Auto-zero techniques, which take the unit off-line for a period of time, are occasionally applied to mask the zero instability inherent with the technology.
Photoacoustic Infrared Technology
The latest innovation for ambient gas monitoring is photoacoustic infrared technology, this builds upon the success of basic infrared technology for percent level or high ppm detection. Photoacoustic infrared technology also exposes the sample of gas to infrared light, but in contrast to absorptive infrared, the reading is based upon what happens to the gas after the infrared light is absorbed. Using this technique a direct gas reading is obtained, this is because a reference sample is not required as a comparison.
In a photoacoustic infrared instrument, a gas sample is introduced into the measurement chamber of the monitor, and the sample is exposed to a specific wavelength of infrared light. If the sample contains the gas of interest, it will take in infrared light proportional to the concentration of gas existing in the sample.
Photoacoustic infrared analysis goes a step further than just calculating how much infrared light is absorbed. It also monitors what happens to the gas after the infrared light is absorbed. In any gas the molecules are constantly moving, and they generate pressure when they move around inside the measurement chamber.
When infrared light is absorbed by a gas, the molecules get hotter, and they begin to move more quickly, resulting in the pressure inside the measurement chamber increasing. The pressure generates an audible pulse that can be identified by an extremely sensitive microphone found in the photoacoustic infrared monitor.
The magnitude of the pressure pulse indicates the concentration of the gas present. The stronger the pressure pulse, the more gas that is detected. The sensitive microphone inside the monitor can identify the smallest of pressure pulses, allowing it to detect even the lowest levels of gas.
The optical filter will only pass the specific wavelength of light for the gas under consideration, so a pressure pulse indicates that the gas is there. If there is no pressure pulse it means that gas is absent, so pressure or heat changes will not affect the units zero measurement.
Furthermore, aging of the IR source or microphone will not affect this zero reading, since the zero is based upon a true zero reading and not the contrast between two readings as in absorptive IR.
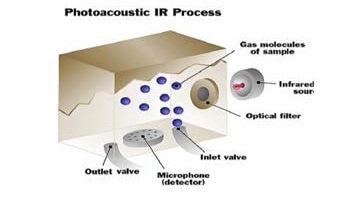
Sample gas enters the measuring cell.
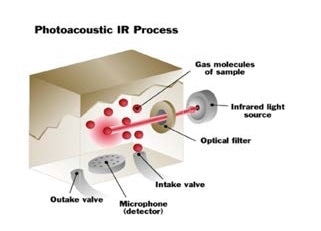
The gas is irradiated with pulsed infrared energy. The pressure changes, as a result of the heating and cooling of the molecules and is measured by the detector.
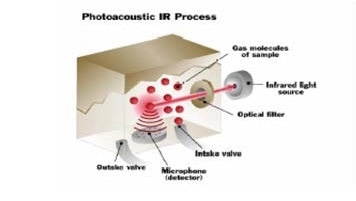
The gas molecules heat and cool as they absorb the infrared energy. The pressure changes, as a result of the heating and cooling of the molecules measured by the detector.
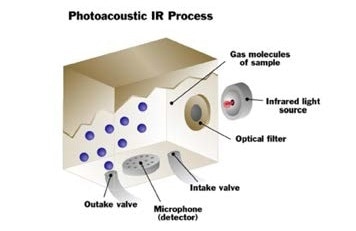
The gas is exhausted and a fresh sample enters the cell. This sampling process is continuously repeated.
The Need for Low-Level Detection
The concentration of airborne substances which workers can be regularly exposed to without adverse health effects is known as the Threshold Limit Value (TLV). The aim of gas-detection apparatus is to verify that gases are identified at concentrations equal to or lower than the TLV, to ensure a safe working environment.
The TLV can be extremely low with certain refrigerant gases, requiring a detection method that can identify that gas at a very low level. The more sensitive an instrument, the lower levels of gases it can detect.
Absorptive infrared monitors can easily identify gases in percentage by high part-per-million (ppm) or volume levels. However, the detection limits for many absorptive infrared monitors can be a lot higher than the TLV of many gases. These instruments need to have longer sample chambers to reach the necessary ppm level of detection, increasing the overall size of the monitor, as well as the cost.
Photoacoustic infrared monitors can detect gases at low ppm, as much as part-per-billion (ppb), levels due to the extreme stability and sensitivity of the microphone that gauges the pressure pulses. This allows photoacoustic infrared monitors to detect the presence of a toxic compound before the concentration reaches the TLV, by detecting tiny pressure pulses, for a wide variety of gases.
Considering Cross-Sensitivity
Cross-sensitivity is a crucial factor to consider when deciding which technology to use to detect refrigerant gases. It is the capability to differentiate between various gases that a single sample may possess. When testing for a refrigerant, it is quite possible that another gas with similar absorption characteristics is present in the chamber.
Even ambient air can cause cross-sensitivity difficulty because of the variability of carbon dioxide or relative humidity in the atmosphere. If a monitor is cross-sensitive to CO2, simply breathing on the monitor can cause a false reading.
Photoacoustic infrared monitors are like other infrared monitors because they use specific optical filters to minimize cross-sensitivity. Considering the use of optical filters and the stability of photoacoustic infrared technology, the sensitivity and selectivity required for low ppm detection can be achieved.
The Need for Zero Stability
Zero stability or preserving a stable baseline is crucial for low ppm detection. If it is not stable low-level detection can be compromised. This can cause false alarms inaccurate readings, and restricted detection levels.
A common problem with absorptive infrared monitors is the fact that the zero acquired from the sample to reference ratio is prone to drift. This is based on a number of factors, including: normal aging, ambient effects, and contamination over time.
As previously explained, it is critical that the balance between the cells is retained, since the absorptive-infrared-monitor reading compares the readings of the sample gas to the reference gas. The monitor must be re-zeroed, if this balance is not maintained, to ensure that the zero point is correct. If not, the monitor may present a false alarm or, worse, be unable to detect the gas in question at low concentrations.
The monitor is not detecting refrigerant gas if autozeroing is used during the rezeroing process. Photoacoustic infrared technology offers zero stability by eliminating the need to alter for zero drift. There is no zero balancing involved — so it provides the most reliable and accurate readings.
Choosing the Right Technology for You —The Photoacoustic Infrared Difference
Photoacoustic infrared monitors are the best choice for installations requiring detection of a refrigerant gas at a very low level, especially in environments where cross sensitivity is an issue. They provide high-performance, low-cost, precise monitoring for a variety of gases.
Currently, the monitors can detect over 20 of the most common refrigerants on the market. These include:
- R11
- R12
- R22
- R123
- R134A
- R401A
- R404A
- R407A
- R407C
- R407F
- R410A
- R422A
- R422D
- R427A
- R507
- R1233zd(E)
- R1234yf
- R1234ze
- R-513A
- R-514A
Photoacoustic infrared monitoring systems can be expanded to sample up to sixteen separate sites.
Choose Wisely
Absorptive infrared monitors are often an appropriate choice in gas detection— particularly when higher detection levels are acceptable.
However, photoacoustic infrared monitors offer the best package of performance and value when the situation calls for an extremely low-level alarm in the presence of other gases and when reliability is critical.
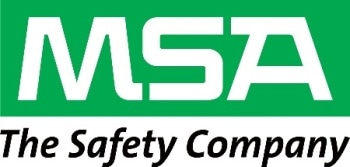
This information has been sourced, reviewed and adapted from materials provided by MSA - The Safety Company.
For more information on this source, please visit MSA - The Safety Company.