Bioelectronics, an emerging branch of science, includes the application of biology and electrical engineering. This article will provide an overview of the integration of sensors into bioelectronics and its application in the field of medicine.
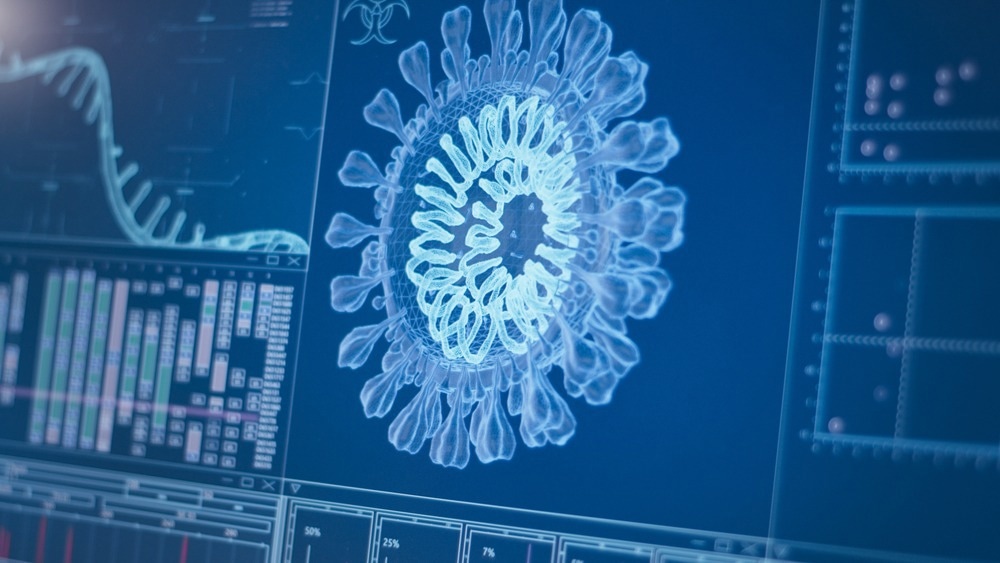
Image Credit: Marcin Janiec/Shutterstock.com
The integration of sensors into electronic systems is a fast-growing area that has the potential to drive research and health. This industry holds a significant role in developing many critical applications in medicine, such as artificial pacemakers and medical imaging.
An Introduction to Bioelectronics and Biosensors
The first electrocardiograph was invented by William Einthoven in 1903 to measure the electrical activity within the heart. The use of bioelectronic devices within the human body has become a revolutionary development for medicine, with these devices being able to accurately detect physiological signals from human bodies. This can aid with diagnoses, health monitoring, therapy as well as advanced treatments.
Bioelectronic devices comprise a biosensor, which works to transform physical phenomena into electrical signals, and this is used to detect changes caused by biological behavior through physical or chemical responses.
Biosensors consist of three components, including a recognition element, a transducer, and an acquisition system. The recognition element is used for sensing targets, while a transducer is utilized to convert chemical reaction signals into analog electrical signals. Additionally, the acquisition system comprises an analytical device, which digitalizes the signal for analysis as well as facilitate easy storage.
Applications of Bioelectronics
The field of bioelectronics aims to merge biological systems with electronic instrumentation; the approach integrates multidisciplinary industries and fields such as material science, chemistry and biology.
Critical areas that have been identified for the integration of biosensors or bioelectronic biosensors include conductive materials such as inherently conductive polymers (ICPs), or conductive carbon nanomaterials. These can be engineered with a range of chemical linkages as well as various-sized structures or utilized with metallic or semiconductor electrode surfaces on electronics.
Another developmental area for biosensor integration comprises incorporating conductive materials into bioresponsive hydrogels for enhanced processing, such as 3D printing. This can also include the use of electroconductive hydrogels onto microelectrodes to construct cell-on-chip technology. Additionally, biorecognition components, including enzymes, are a biosensor developmental area that can enhance selectivity for target analytes.
The most recent application of biosensors consists of an artificial retina, which comprises an implantable image sensor. This application is significant for treating eye diseases and disorders, including retinitis pigmentosa and aged macular degeneration.
These disorders can cause the loss of function of photoreceptor cells; however, ganglion cells, the most inner retinal neurons, have been found to be histologically healthy and intact. This is critical as it means the healthy retinal cells that remain intact can be stimulated to create retinal prosthesis vision, which would be revolutionary for the bioelectronics field as well as individuals suffering from vision loss due to these conditions.
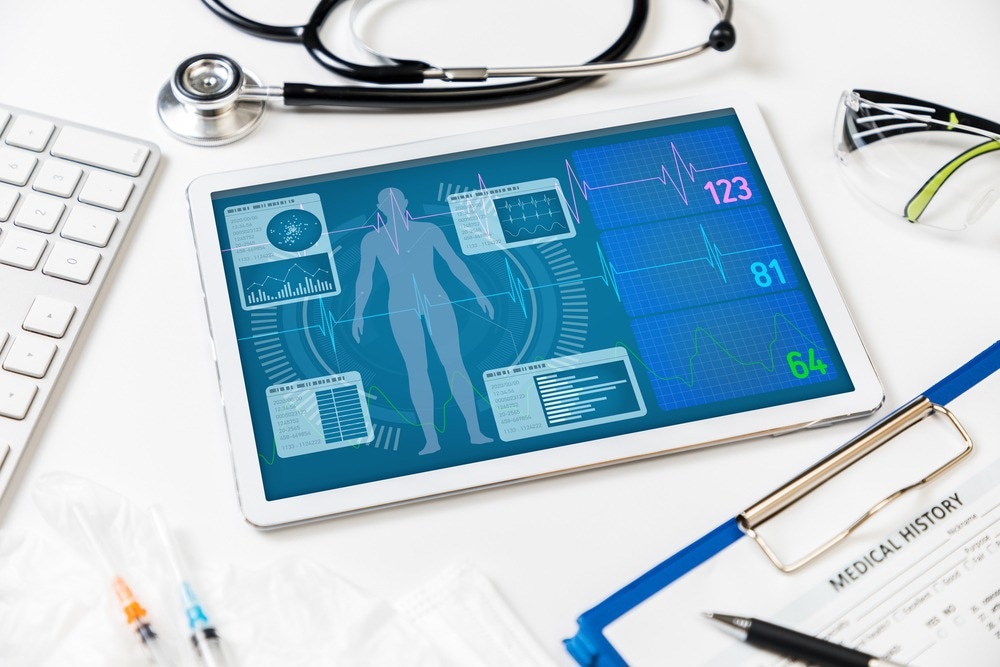
Image Credit: metamorworks/Shutterstock.com
Benefits and Challenges
The benefit of integrating biosensors into bioelectronics includes the characteristic of being highly selective, which can enable tailoring specific interactions using recognition elements.
There is currently an increased number of sensors that are being incorporated into diagnostic tools, such as for point-of-care testing. A significant example of this includes glucose sensors that are used to detect glucose levels for those that require monitoring, such as diabetic patients.
The enhancement of point-of-care testing devices with biosensors can aid in its highly selective nature, which can be critical for the early detection of clinical biomarkers – this can assist with predicting the onset of potential diseases.
The development of these devices, which can be miniature, fast, and effective without requiring a laboratory, can be beneficial for a wide population as well as for a variety of health conditions.
However, challenges in this area include satisfying all these criteria and creating a sensing device that is miniature, practical as well as cost-effective. Producing this type of device can be high in cost due to the various components with which it is constructed, including surface functionalization to enhance selectivity towards analytes.
Future Outlook
Incorporating sensors into bioelectronics can be significant for the advancement of many medical devices, such as the use of artificial pacemakers, as well as point-of-care testing devices. This advancement in bioelectronics is revolutionary for the field of medicine and healthcare due to prioritizing treating patients with various diseases and disorders.
Using biosensor point-of-care testing devices that are miniature, effective and with fast results can allow patients to monitor their diseases in real-time, such as clinical biomarkers for cardiovascular diseases as well as even cancer. This can ensure patients have more interaction with their health and aid them in taking a more personal approach to their treatment plan. This may help them to follow treatments more effectively, see their doctors when concerns arise, and overall assist with early disease detection and treatment management.
Furthermore, the integration of biosensors with bioelectronics can also aid with developing advanced biotechnologies, such as an artificial retina, which can help with vision loss for patients suffering from degeneration of photoreceptors in the eye.
The combination of sensors and bioelectronics holds limitless potential for the advancement of both the bioelectronics industry as well as for medicine and healthcare, which may aid in solving critical challenges faced by populations worldwide.
References and Further Reading
Aggas, J.R. et al. (2021) “On the intersection of molecular bioelectronics and biosensors: 20 years of c3b,” Biosensors and Bioelectronics, 176, p. 112889. doi.org/10.1016/j.bios.2020.112889.
Chan, S.-H. et al. (2016) “Integration of bioelectronics and bioinformatics: Future direction of bioengineering research,” Journal of Medical and Biological Engineering, 36(6), pp. 751–754. doi.org/10.1007/s40846-016-0185-1.
Grieshaber, D. et al. (2008) “Electrochemical biosensors - sensor principles and Architectures,” Sensors, 8(3), pp. 1400–1458. doi.org/10.3390/s80314000.
Omidfar, K. et al. (2020) “Point-of-care biosensors in medicine: A brief overview of our achievements in this field based on the conducted research in EMRI (Endocrinology and Metabolism Research Institute of Tehran University of Medical Sciences) over the past fourteen years,” Journal of Diabetes & Metabolic Disorders [Preprint]. doi.org/10.1007/s40200-020-00668-0.
Yu, Y. et al. (2020) “Flexible electronics: Flexible electrochemical bioelectronics: The rise of in situ bioanalysis (adv. mater. 15/2020),” Advanced Materials, 32(15), p. 2070115. doi.org/10.1002/adma.202070115.
Disclaimer: The views expressed here are those of the author expressed in their private capacity and do not necessarily represent the views of AZoM.com Limited T/A AZoNetwork the owner and operator of this website. This disclaimer forms part of the Terms and conditions of use of this website.