AZoSensors speaks with Professor Regina Ragan about the development of a new water monitoring technology that utilizes the bacterium E.coli to create a live sensor. We discuss how this sensor works and why heavy metal contamination is so important to public health strategies.
Please could you introduce yourself and your current research activities?
I am a Professor of Material Science and Engineering. My research interests span gaining a fundamental understanding of physicochemical driving forces in self-assembly to translating developed nanomaterials into environmental sensors and medical diagnostic devices, which simultaneously offer low-cost and record performance.
How did you become involved in this field of research?
I have always been motivated to pursue scientific research leading to developing devices with societal benefits. Thus, I have focused on areas where fundamental studies can fill knowledge gaps to generate devices benefiting the health of people and the environment. Nanomaterials offer unprecedented device performance and self-assembly offers the ability to manufacture at scale and with low cost.
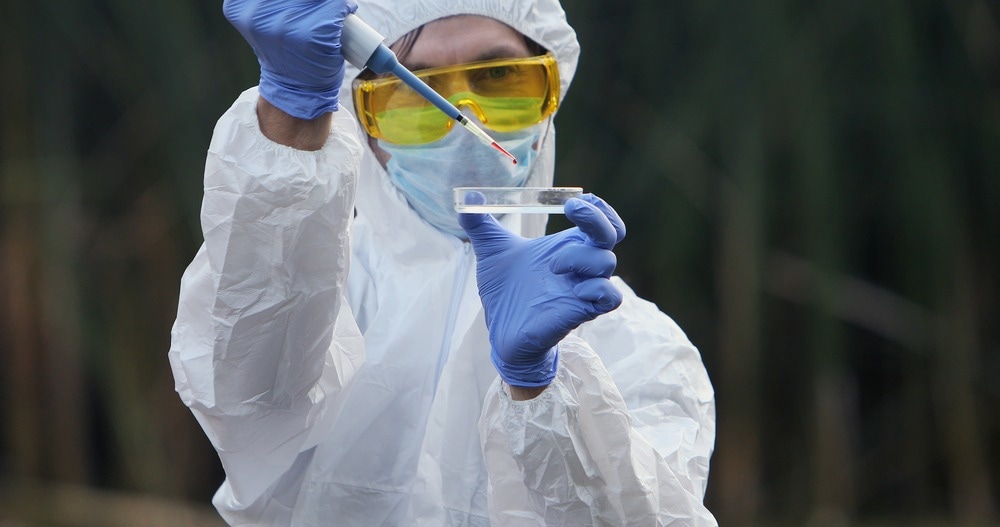
Image Credit: Creadores de Video/Shutterstock.com
What are the risks associated with heavy metal contamination in water?
Contaminated water is the main source of metal toxin exposure to people and animals. Heavy metal toxins can lead to diseases including cancer and diabetes and accumulate in organs disrupting normal biological function.
Why do bacteria like E. coli present an interesting platform for sensing trace elements and chemicals?
E. coli is a model organism for metabolic engineering and the stress response has been extensively studied. We were able to confirm our hypothesis that the cascade of chemical signals detected by our sensors could be ‘decoded’ with machine learning algorithms.
Could you discuss the newly developed E. coli-based water monitoring system?
The metabolic stress response of E. coli has differing chemical signatures from different metal toxins and varies with exposure concentration. Thus, analysis of this data allows us to quantify metal type and concentration.
How does this system compare to existing technologies?
The system’s limit of detection and quantification compares with inductively coupled plasma-mass spectrometry, which requires complex infrastructure and a cost in excess of $200,000. Our developed sensors be used with a portable, tabletop spectrometer, which costs approximately $1000.
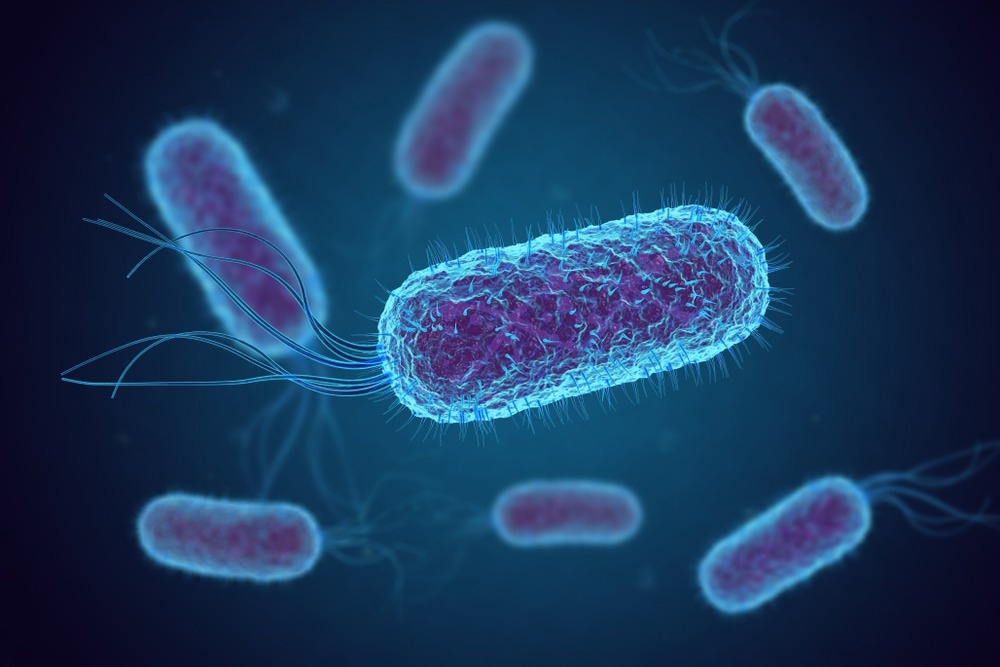
Image Credit: fusebulb/Shutterstock.com
What key technical components, such as the use of gold nanoparticles, enabled the system to have such a high specificity and speed of detection?
The self-assembly process defining surface chemistry and inter-particle spacing of gold nanoparticles, developed by Ragan, produces billion-fold signal enhancements that allow us to reach ultralow detection limits.
What role does the algorithm play in the water monitoring system?
The algorithm is used to understand the complex cascade of chemical signals detected and is analogous to recognizing a fingerprint for individual identification. As a result, the algorithm enables automated analysis.
What are the next steps for this investigation?
In the future, we hope to demonstrate that the technology can be used to detect other metal toxins.
About Professor Regina Ragan
Prof. Regina Ragan is a Professor in the Department of Material Science and Engineering and co-PI of the Center for Complex and Active Materials, a NSF MRSEC at UC Irvine. She has served as Equity Advisor, Inclusive Excellence Professor, and Diversity Director in Engineering Education. She is a recipient of the National Science Foundation Faculty Early CAREER Award and a Fulbright Fellow. Before joining UC Irvine, she received her B.S. summa cum laude in Material Science and Engineering from the University of California, Los Angeles, Ph.D. in Applied Physics from the California Institute of Technology. She was a postdoctoral scholar in the Information & Quantum Systems Laboratory at Hewlett Packard.
Since joining the Faculty at UC Irvine, she began a research effort in self-assembly as she foresaw this would play a vital role in (nano) manufacturing of nanoscale devices and pioneered methods for assembling colloidal metal nanoparticles into photonic devices, a nascent research area a decade ago. Her activities include investigating and gaining fundamental understanding material platforms capable of assembling at scale, integration in device architectures, and connecting architecture design with device performance.
Disclaimer: The views expressed here are those of the interviewee and do not necessarily represent the views of AZoM.com Limited (T/A) AZoNetwork, the owner and operator of this website. This disclaimer forms part of the Terms and Conditions of use of this website.