Optical gas sensors play a crucial role in various sectors, utilizing techniques like absorption, fluorescence, and laser spectroscopy to detect and quantify gases. With a market expected to grow to USD 2.3 billion by 2028, these sensors offer real-time monitoring and remote sensing capabilities, facilitating applications ranging from environmental monitoring to industrial processes. Explore further to learn about the working principles, latest innovations, and wide-ranging applications of optical gas sensors.
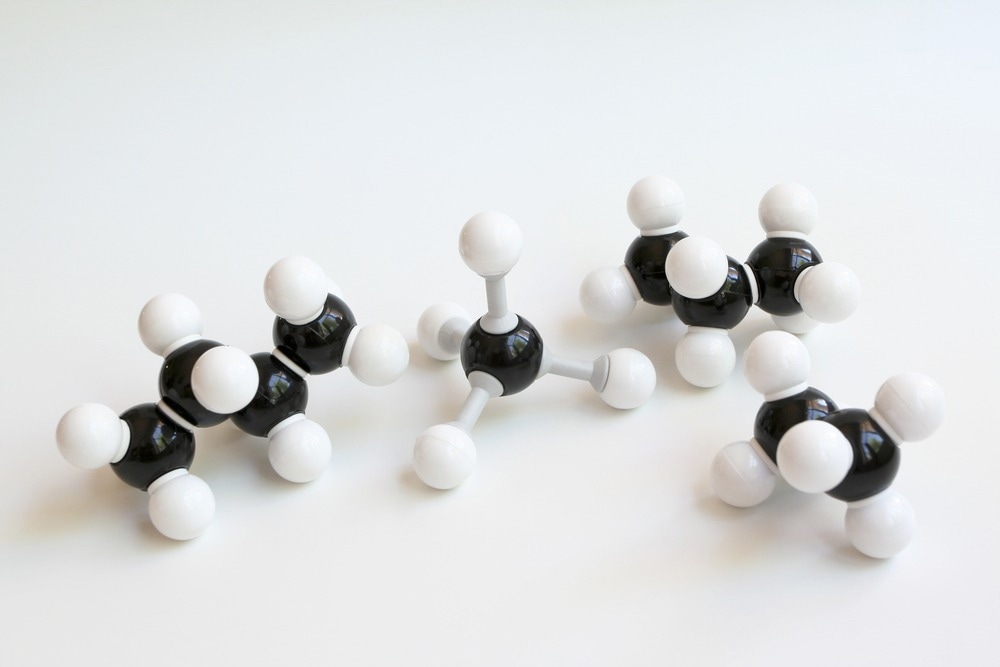
Image Credit: JeffreyRasmussen/Shutterstock.com
Working Principle of Optical Gas Sensors
The main principle of optical gas sensors is based on analyzing the interaction between gaseous components and light. The interaction between light and gaseous species leads to changes in light’s properties, such as scattering, absorption, or emission. These changes are measured by optical gas sensors, which enable the detection of the presence of specific gases and their concentration.
The key factors that influence the effectiveness of optical gas sensing techniques are sensitivity to the lowest volume concentration of target gas, selectivity, response time, stability, adsorption capacity, energy consumption, and manufacturing cost. Most optical gas sensors are based on absorption spectroscopy, fluorescence spectroscopy, and laser-based techniques. These are briefly discussed below.
Absorption Spectroscopy
Absorption spectroscopy is one of the most commonly used techniques in optical gas sensing, which determines light absorption by gaseous species. Absorption spectroscopy is based on the Beer-Lambert law. This law entails the existence of a linear relationship between light absorption and gas concentration. The amount of light absorbed by a gas is directly proportional to its concentration of gas molecules. Therefore, the gas sensors can detect and quantify a particular gas based on light intensity on a particular wavelength.2
Several important organic and inorganic molecules have characteristic absorption properties in the mid-infrared (MIR) spectral region (λ ∼ 2–20 μm). Compared to different types of absorption gas sensors, MIR-based optical gas sensors are highly sensitive and are used in environmental monitoring, medical diagnoses, and industrial processes.3
Optical gas sensors based on absorption spectroscopy are used to detect ozone, benzene, ethylbenzene, nitrogen dioxide, sulfur dioxide, toluene, and xylenes. Detection of these gases is important for all living organisms and the environment. For instance, ozone is a pollutant in the lower troposphere, and it adversely affects humans and the ecosystem. Similarly, nitrogen dioxide is another harmful air pollutant whose primary source is automobile exhaust and the combustion of fossil fuels. Prolonged exposure to nitrogen dioxide can cause inflammation of respiratory tracts, which might damage the lungs.
OHC-800 manufactured by Riken Keiki Co. is an explosion-proof gas calorimeter used in many industrial settings, such as the steel industry, gas companies, and glass manufacturers.4 VIGO Photonics has developed optical gas detectors for leak detection of many common flammable and toxic gases, such as methane.5
Fluorescence Spectroscopy
In fluorescence spectroscopy, a light beam is focused on gas molecules, which absorb photons and get excited. On returning to the ground state, the gas molecules emit fluorescent light at a specific wavelength. An optical gas sensor detects fluorescence emission to determine the presence of a particular gas. This technique is mainly used to detect trace gases.6
A fluorescence spectroscopy-based carbon dioxide gas sensor has been developed to monitor fermentation off-gas production. Organic fluorescence sensors offer higher sensitivity due to reduced background noise. These sensors can detect even a small amount of nitrogen dioxide, and nitroaromatic, propanol, and ethanol vapors. The SG870 oxygen gas sensor, manufactured by Duomo (UK), is based on optical fluorescence technology. This sensor can accurately detect oxygen levels, even in low-oxygen environments.
Laser Spectroscopy
The underlying principle behind laser-based optical gas sensors is the absorbance of light energy by gas molecules at a particular wavelength, and subsequent generation of molecular vibrations. Gas molecules can absorb many light wavelengths and generate absorption lines. When highly monochromatic and coherent light sources are introduced as laser light, the gas molecules, whose emission wavelength is similar to that of the absorption line of the target, dip around the absorption line. This dip helps determine the type and concentration of the target gas.
The two most popular laser-based techniques used in optical gas sensors are cavity ring-down spectroscopy (CRDS) and tunable diode laser absorption spectroscopy (TDLAS).7 The CW Quantum cascade lasers L1200X series, manufactured by Hamamatsu Corporation, is the latest TDLAS that is used to detect ozone, nitrogen dioxide, carbon dioxide, methane, and many other harmful gases.
An optical non-dispersive infrared (NDIR) E-nose has been recently developed that contains an array of four tuneable detectors that can scan a range of wavelengths. This E-nose was found to be sensitive to different gases, such as carbon dioxide and methane, at varied levels, even when they are part of complex mixtures. Currently, optical E-nose technology is rapidly progressing with increased applications.8 E-noses manufactured by Sensifi are used to detect food contaminants.
Latest Innovations in Optical Gas Sensors
In the past two decades, the development of new light sources and detection techniques has revolutionized the application of optical gas sensors. Some of the newly developed light sources are light-emitting diodes (LEDs), quantum cascade lasers (QCLs), and micro-electro-mechanical systems (MEMS)-based thermal emitters.9
Miniaturization of optical devices has increased the reach of optical gas sensors. For instance, these can now be applied to wearable smartphones, tablets, and medical devices. Highly compact and reliable optical gas sensors can be used for food quality control, breath analysis, body fluid and tissue analysis, indoor air quality (IAQ) control, and contamination identification.
Recently, deep learning methods have been implemented for TDLAS gas sensors. This strategy alleviates the problem of spectral cross-interference while allowing simultaneous measurement of multi-component gases.10 Convoluted neural networks (CNN), multilayer perceptron (MLP), and autoencoder (AE) are commonly used ML models in optical gas sensor applications.
Optical fiber gas sensors are typically more dynamic than non-fiber sensors and have wide-ranging applications. Recently, scientists have developed optical fiber gas sensors that can simultaneously detect the presence of formic acid gas, humidity, and temperature. These are highly integrated sensors that exhibit extraordinary sensitivity, anti-interference, selectivity, and safety.11
Micro- and nano-structured optical fibers have been designed to detect parts-per-million to parts-per-trillion levels of gases, such as methane, ethane, carbon monoxide, hydrogen, and oxygen.12 Zinc oxide nanoparticle-coated fiber-optic sensors have been developed based on a plasmon resonance mechanism to detect ammonia, acetone, and ethanol at trace levels.
Analysis of exhaled breath samples helps diagnose many diseases and physiological conditions, such as asthma, diabetes, and infections. For instance, the breath of a patient with cystic fibrosis (CF) contains hydrogen cyanide. This gas is released during the metabolism of Pseudomonas aeruginosa bacteria present in the lungs of CF patients.13
A photoacoustic sensor is a type of optical gas sensor that is used to detect leaks in storage or industrial facilities. It is also used to monitor air quality and disease diagnostics. Quartz-enhanced photoacoustic Sensors (QEPAS) are another type of optical gas sensor that is used to trace gas detection, industrial process control, and greenhouse gas monitoring.15 Integrated optical sensors are miniaturized devices containing all essential optical gas sensing components.15 These sensors offer portability and can be inserted into wearable devices.
References and Further Reading
- Mishra V, Rashmi and Sukriti. Optical Gas Sensors. Metal-Oxide Gas Sensors. IntechOpen. 2023; http://dx.doi.org/10.5772/intechopen.108971.
- Hong S, Wu M, Hong Y, Jeong Y, Jung G, Shin W, Park J, Kim D, Jang D, Lee J. FET-type gas sensors: A review. Sens Actuators B Chem. 2021; 330, 129240. https://doi.org/10.1016/j.snb.2020.129240
- Ng W, Minasny B, Jeon SH, McBratney A. Mid-infrared spectroscopy for accurate measurement of an extensive set of soil properties for assessing soil functions. Soil Security.2022; 6, 100043. https://doi.org/10.1016/j.soisec.2022.100043
- Explosion proof gas colorimeter. Riken Keiki Co. https://product.rikenkeiki.co.jp/lp/en/ohc-800/?creative=658887487665&keyword=natural%20gas%20sensor&matchtype=p&network=g&device=c&gad_source=1&gclid=Cj0KCQiA-62tBhDSARIsAO7twbYeetprDuuy6nu9DH8e6Vq-Fyg3g8hsRUXr9VjoYQkeIIbYYoAX2nIaAhf6EALw_wcB. Assessed on January 20, 2024.
- Advanced Gas Leak Detectors: Infrared and Gas Detectors. Vigo Photonics. https://vigophotonics.com/applications/laser-gas-leak-detection-with-infrared. Assessed on January 20, 2024.
- Wang J, Wen Z, Yang B, Yang X. Optical carbon dioxide sensor based on fluorescent capillary array. Results Phys. 2017; 7, 323-326. https://doi.org/10.1016/j.rinp.2016.12.044
- Gong W, Hu J, Wang Z, Wei Y, Li Y, Zhang T, Zhang Q, Liu T, Ning Y, Zhang W, Grattan KT. Recent advances in laser gas sensors for applications to safety monitoring in intelligent coal mines. Front Phys. 2022. 10, 1058475. https://doi.org/10.3389/fphy.2022.1058475
- Esfahani S. et al. Development of a Tuneable NDIR Optical Electronic Nose. Sensors. 2020; 20, 6875; doi:10.3390/s20236875.
- Popa D, Udrea F. Towards Integrated Mid-Infrared Gas Sensors. Sensors. 2019; 19(9), 2076. https://doi.org/10.3390/s19092076
- Sun J, Chang J, Wei Y, Zhang Z, Lin S, Wang F, Zhang Q. Dual gas sensor with innovative signal analysis based on neural network. Sens Actuators B Chem. 2022; 373, 132697. https://doi.org/10.1016/j.snb.2022.132697
- Chen G, Li J, Zhu H, Wang Y, Ji H, Meng F. Optical fiber gas sensor with multi-parameter sensing and environmental anti-interference performance. J. Ind. Inf. Integr. 2024; 100565. https://doi.org/10.1016/j.jii.2024.100565
- Jin W, Bao H, Zhao P. et al. Recent Advances in Spectroscopic Gas Sensing With Micro/Nano-Structured Optical Fibers. Photonic Sens. 2021; 11, 141–157. https://doi.org/10.1007/s13320-021-0627-4
- Rosa RL, Johansen HK, Molin S. Adapting to the Airways: Metabolic Requirements of Pseudomonas aeruginosa during the Infection of Cystic Fibrosis Patients. Metabolites. 2019; 9(10). https://doi.org/10.3390/metabo9100234
- Liu Y, Lin H, Montano BAZ, Zhu W, Zhong Y, Kan R, Yuan B, Yu J, Shao M, Zheng H. Integrated near-infrared QEPAS sensor based on a 28 kHz quartz tuning fork for online monitoring of CO2 in the greenhouse. Photoacoustics.2022; 25, 100332. https://doi.org/10.1016/j.pacs.2022.100332
- Brandenburg A, Edelhaäuser R, and Hutter F. Integrated optical gas sensors using organically modified silicates as sensitive films. Sens Actuators B Chem. 1993; 11(1-3), 361-374. https://doi.org/10.1016/0925-4005(93)85276-G
Disclaimer: The views expressed here are those of the author expressed in their private capacity and do not necessarily represent the views of AZoM.com Limited T/A AZoNetwork the owner and operator of this website. This disclaimer forms part of the Terms and conditions of use of this website.