By Ankit SinghReviewed by Susha Cheriyedath, M.Sc.Oct 6 2024
Chemical sensors are essential tools across numerous industries due to their ability to detect and analyze chemical data. These devices are specifically engineered to transform chemical information, such as analyte concentrations, into measurable signals that enable real-time analysis
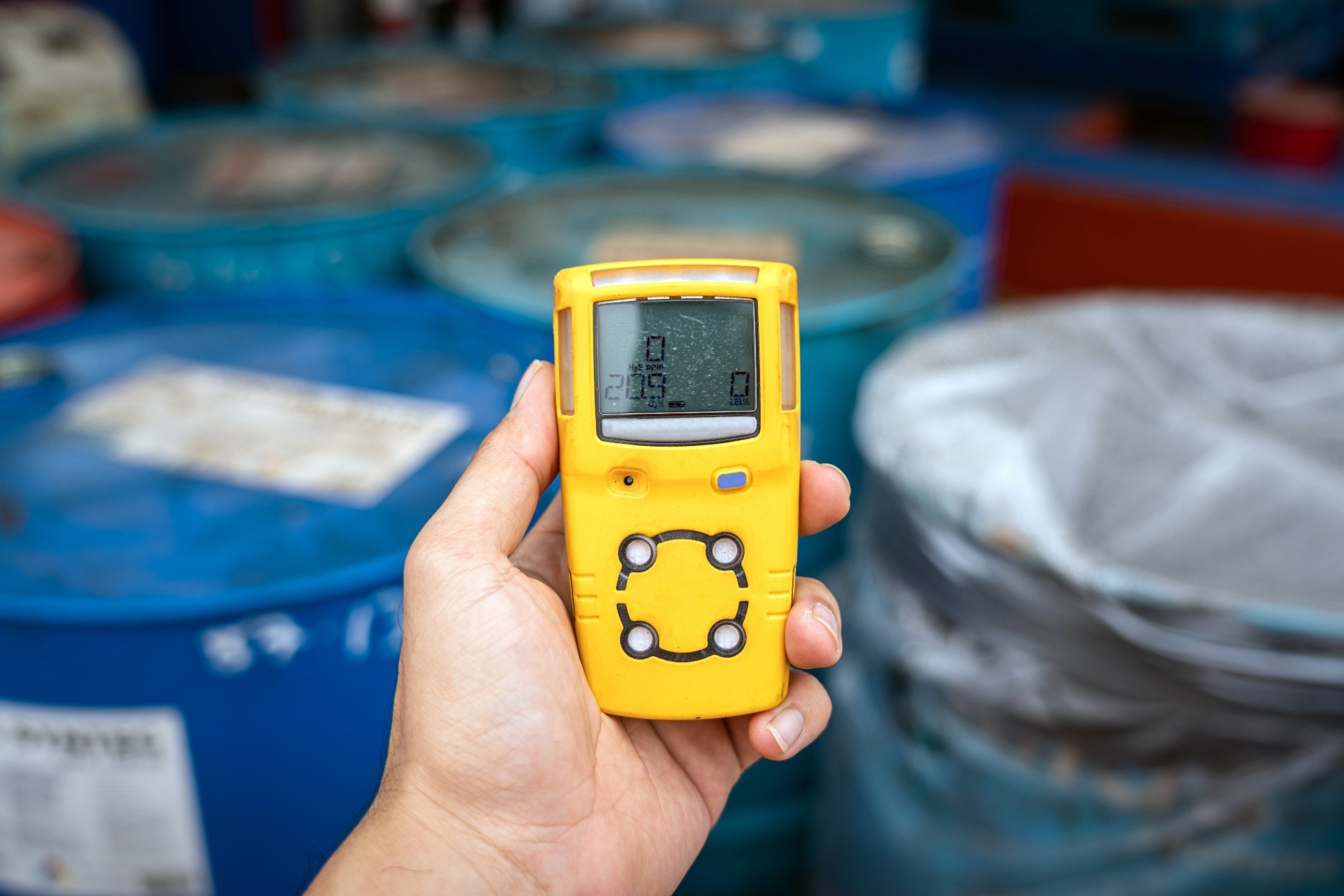
Image Credit: Nattawit Khomsanit/Shutterstock.com
From environmental surveillance to healthcare diagnostics, chemical sensors play a vital role in improving safety, optimizing efficiency, and driving technological innovation. The increasing demand for rapid, cost-effective, and highly sensitive detection systems has sparked extensive research and advancements in the field.
Structure and Components of Chemical Sensors
At the heart of every chemical sensor lies a combination of key components that work in tandem to detect specific analytes. The most crucial of these is the sensing element, which interacts directly with the target analyte, such as gases, ions, or molecules. The materials chosen for the sensing element depend on their selectivity and reactivity to the substance being measured. Commonly used materials include nanomaterials, polymers, metal oxides, and biological molecules. For instance, metal oxide-based sensors are widely applied in gas detection due to their high surface reactivity.1
The transducer converts the chemical interaction between the analyte and the sensing element into a measurable signal. This signal can be electrical, optical, or thermal, depending on the sensor design. The efficiency of the transducer is crucial as it affects the sensor's sensitivity and response time. For example, electrochemical sensors monitor changes in current or voltage, while optical sensors track variations in light intensity.
Once the transducer generates a raw signal, it is processed by the signal processor, which amplifies and converts the data into a format that can be easily interpreted. Modern chemical sensors often include microprocessors that filter out noise and provide a digital output, improving accuracy, especially when detecting low concentrations of an analyte.
Lastly, the supporting structures of a chemical sensor provide mechanical stability and protect it from environmental factors like humidity or dust. In miniaturized or wearable sensors, these structures are often lightweight and flexible while maintaining durability to function reliably under various conditions.1
By integrating these components, chemical sensors can efficiently detect a wide array of analytes, ranging from industrial gases to biomolecules.
What Are the Different Types of Chemical Sensors?
Chemical sensors can be categorized based on the mechanism by which they convert chemical information into signals. These include electrochemical, optical, thermal, piezoelectric, and biosensors. Each type of sensor operates using a distinct transduction method, making them suitable for different applications.
Electrochemical sensors detect electrical changes resulting from chemical interactions. These sensors are known for their high sensitivity, but they may suffer from cross-sensitivity, where unwanted substances interfere with measurements. Calibration and sensor design play key roles in mitigating such interference.2,3
Optical sensors detect changes in light properties, such as absorption or fluorescence, and offer high selectivity due to their ability to distinguish between different wavelengths of light. These sensors often provide a large dynamic range, allowing them to detect both low and high concentrations of analytes with precision.
Thermal sensors measure temperature changes from chemical reactions. Response time and recovery time are important factors in the performance of thermal sensors, making them ideal for applications where reaction heat needs to be monitored quickly and accurately.
Piezoelectric sensors detect changes in mechanical stress or mass, with applications like Quartz Crystal Microbalance (QCM) sensors being used for ultra-sensitive biosensing by detecting minute mass changes through frequency shifts.
Finally, biosensors combine biological recognition elements such as enzymes, antibodies, or DNA with a transducer to detect specific biological analytes. These sensors are particularly useful in medical diagnostics, and they often require regular calibration to maintain precision.4
Key Technical Considerations in Chemical Sensor Design
The performance of chemical sensors hinges on several key technical factors that determine their effectiveness in detecting and analyzing chemical substances.
Sensitivity and selectivity are crucial to how well these sensors function. Sensitivity refers to the lowest concentration of a chemical analyte that a sensor can reliably detect, a vital feature in applications where trace amounts of a substance need to be identified, such as in environmental monitoring or healthcare diagnostics.
Selectivity, in contrast, measures the sensor’s ability to differentiate between the target chemical analyte and other potential substances in the sample environment. Advances in nanomaterials, such as carbon nanotubes and graphene, have significantly improved both sensitivity and selectivity. These materials offer a much larger surface area for chemical interaction, allowing the sensor to detect minute quantities of specific substances with greater precision.
Calibration is another critical component of chemical sensor performance. Over time, the accuracy of a sensor can degrade due to environmental factors, sensor aging, or contamination, resulting in signal drift. For chemical sensors, particularly those used in continuous monitoring environments like industrial processes or medical diagnostics, maintaining consistent accuracy is paramount. Calibration techniques are employed to minimize signal drift, ensuring that the sensor provides reliable data throughout its operational life.
Another essential factor is the sensor's dynamic range, which refers to the range of chemical concentrations it can accurately measure. In many chemical applications, sensors need to detect both very low and very high concentrations of a chemical analyte without sacrificing accuracy. For example, in environmental pollution monitoring, sensors must be able to detect trace amounts of harmful gases as well as higher concentrations in industrial settings. Extending the dynamic range of chemical sensors is thus a key focus of sensor design, enabling them to perform effectively across diverse applications.
Interference and cross-sensitivity are common challenges in chemical sensor usage, particularly when operating in complex chemical environments where multiple substances are present. Chemical sensors can sometimes respond to substances other than the target analyte, leading to inaccurate or noisy data. This issue, known as cross-sensitivity, can be mitigated by using selective sensor coatings, filtration systems, and sophisticated signal processing techniques designed specifically for chemical detection. By improving the ability of chemical sensors to ignore non-target substances, engineers can enhance their performance in real-world applications where background interference is a concern.
The design and fabrication of chemical sensors also play an important role in their functionality. Techniques such as thin-film deposition, chemical vapor deposition, and microfabrication allow for the creation of sensors that are not only highly sensitive but also small and energy-efficient. This is key in modern applications like wearable chemical sensors for healthcare or portable environmental monitoring devices, where sensors must be lightweight, durable, and power-efficient. Advances in these fabrication methods enable the development of chemical sensors that meet the growing demand for smaller, more efficient, and more reliable detection systems.
Applications of Chemical Sensors
Chemical sensors have a vast range of applications across various sectors:
- Environmental Monitoring: For detecting pollutants and ensuring compliance with regulations.
- Healthcare: Biosensors in glucose monitoring, pathogen detection, and diagnostic tests.
- Industrial Processes: Ensuring chemical safety and product quality control.
- Food Safety: Monitoring contamination and spoilage in food production.4
Recent Trends in Chemical Sensors
Recent advancements in material science and nanotechnology have significantly boosted the capabilities of chemical sensors. Nanomaterials such as carbon nanotubes, graphene, and gold nanoparticles have been instrumental in improving sensor sensitivity and selectivity. These materials provide a larger surface area for analyte interactions, allowing for the detection of extremely low concentrations. For instance, a review published in Sensors demonstrated the efficacy of nanostructured materials in detecting toxic metal ions like lead, mercury, and cadmium, greatly enhancing both sensitivity and target selectivity.5
Wearable chemical sensors are gaining traction, particularly in healthcare. Embedded in devices such as smart patches, these sensors continuously monitor vital biomarkers, like glucose levels and electrolytes, offering real-time health monitoring. In addition, advancements in toxic material detection are focusing on portability and accuracy, with innovations that combine multiple sensing techniques for enhanced performance in complex environments.5
The Sensors Industry: A Comprehensive Guide
Market Trends and Competition
The chemical sensor market is highly competitive, with manufacturers focusing on improving sensitivity, reducing response times, and miniaturizing sensors for portable and wearable applications. Nanotechnology is playing a critical role in these improvements, enabling the creation of sensors that are faster and more sensitive to low analyte concentrations. Companies like Honeywell and Figaro are leaders in incorporating nanomaterials to elevate sensor performance.
In parallel, the development of wearable sensors and their integration with the Internet of Things (IoT) is transforming sectors like healthcare and industrial safety. Companies such as Abbott Laboratories and Sensirion are pioneering the use of wearable sensors that enable continuous, remote monitoring for health management and environmental applications.
Artificial intelligence (AI) and machine learning are also reshaping the chemical sensor industry. Companies like Siemens are embedding AI into sensor systems to enhance data analysis, improve predictive maintenance, and increase the accuracy of multi-analyte detection, making sensors smarter and more efficient across various applications.
Future Prospects and Conclusion
The future of chemical sensors is promising, with ongoing research aiming to further improve sensitivity, reduce response times, and enhance multi-analyte detection capabilities. The integration of AI and machine learning is expected to play a pivotal role in optimizing sensor performance, particularly in predictive monitoring and advanced data analysis.
Moreover, with a growing emphasis on environmental sustainability, the development of eco-friendly and biodegradable materials for chemical sensors is becoming a key area of focus. This shift will help reduce the environmental footprint of sensor production and disposal, aligning with global sustainability goals.
As chemical sensors continue to evolve, they will drive advancements in healthcare, environmental protection, and industrial efficiency. These sensors will remain integral to ensuring safety, improving quality control, and enabling real-time monitoring in increasingly complex environments.
Advancements in Sensor Technology: What to Expect by 2030
References and Further Reading
- Mohindru, P. (2023). A review on smart sensors used in chemical industry 4.0. Journal of Data Acquisition and Processing. Vol. 38 (2). 1172. DOI:10.5281/zenodo.776698. https://www.researchgate.net/publication/373093137_A_REVIEW_ON_SMART_SENSORS_USED_IN_CHEMICAL_INDUSTRY_40
- Saputra, H.A. (2023). Electrochemical sensors: basic principles, engineering, and state of the art. Monatsh Chem 154, 1083–1100. DOI:10.1007/s00706-023-03113-z. https://link.springer.com/article/10.1007/s00706-023-03113-z
- Baranwal, J. et al. (2022). Electrochemical Sensors and Their Applications: A Review. Chemosensors, 10(9), 363. DOI:10.3390/chemosensors10090363. https://www.mdpi.com/2227-9040/10/9/363
- Chen, X. et al. (2022). Microarray-based chemical sensors and biosensors: Fundamentals and food safety applications. TrAC Trends in Analytical Chemistry, 158, 116785. DOI:10.1016/j.trac.2022.116785. https://www.sciencedirect.com/science/article/abs/pii/S0165993622002680
- Kim, Y. et al. (2023). Recent Trends in Chemical Sensors for Detecting Toxic Materials. Sensors, 24(2), 431. DOI:10.3390/s24020431. https://www.mdpi.com/1424-8220/24/2/431
Disclaimer: The views expressed here are those of the author expressed in their private capacity and do not necessarily represent the views of AZoM.com Limited T/A AZoNetwork the owner and operator of this website. This disclaimer forms part of the Terms and conditions of use of this website.