Sep 22 2017
Shafigh Nategh, while conducting research as a Graduate Student at KTH School of Electrical Engineering, Stockholm, Sweden, demonstrated the ability of high thermal conductivity materials to advance performance in electric machines.
Nategh’s research, “Thermal Analysis and Management of High-Performance Electrical Machines,” focused on thermal management aspects of electric machinery employed in high-performance applications, with specific focus on electric motors designed for hybrid electric vehicle applications.
In Nategh’s research, LORD CoolTherm™ SC-320 Thermally Conductive Silicone Encapsulant, a relatively soft, high thermal conductivity material (3.2 W/m•K) with adequately low viscosity to be used in vacuum potting was evaluated. The electrically-insulating silicone encapsulant, designed for electrical/electronic applications, offers exceptional thermal conductivity properties while retaining the desirable properties related to silicones.
CoolTherm SC-320 encapsulant is a two-component system that shows low shrinkage and stress on components as it cures and maintains a low viscosity for ease of component encapsulation. Environmentally resistant and UL-rated to UL94V0 and 180C RTI, CoolTherm SC-320 encapsulant is made up of an addition-curing polydimethyl siloxane polymer that does not get depolymerized when heated in confined spaces.
In the first part of Nategh’s thesis, new thermal models of liquid-cooled (water and oil) electric machines (that is, motors) were proposed based on a combination of lumped parameter (LP) and numerical methods. A permanent-magnet assisted synchronous reluctance machine (PMaSRM) provided with a housing water jacket, as well as an oil-cooled induction motor where the oil was in direct contact with the stator laminations, were analyzed. In the second part of the thesis, the thermal impact of using varied winding impregnation and steel lamination materials was assessed.
Standard varnish and epoxy, as well as a silicone-based thermally conductive impregnation material (CoolTherm SC-320 encapsulant), were studied followed by a comparison of the resulting temperature distributions in three small permanent magnet motors.
In comparing the effects of different impregnation materials, the hot spot temperatures of the windings were measured under different coolant flow rates and present levels for each of the potted motors. The hot spot temperatures of the motor impregnated with CoolTherm SC-320 encapsulant were usually 40 °C to 45 °C cooler than the varnish-only motor, and about 12 °C to 15 °C cooler than the epoxy-potted motor.
With CoolTherm SC-320 encapsulant, no difficulties in vacuum filling were noted, despite its viscosity being rather higher than those of the epoxy and varnish. In addition, the potting compound played an effective role in decreasing the hot spot temperatures even when the potting was not 100% dense. For instance, the hot-spot temperature of motors potted with CoolTherm SC-320 encapsulant increased by just 3 °C as the potting density was decreased from 80% to 50%, while the hot spot temperatures of epoxy and varnish-potted motors increased by 19 °C and 25 °C, respectively, as their potting densities were lowered from 80% to 50%.
Nategh’s research demonstrated that hot spot temperatures can be decreased by 35% to 50% using CoolTherm SC- 320 encapsulant as compared to an unpotted motor, compared to advancements of only 20% to 30% using typical epoxy potting materials. The potting compound may provide major improvements in power density of electric motors.
A decrease in the hot-spot temperature of motors, based on current, is capable of providing an increase in achievable torque/power for a given motor size, decrease in motor size for a desired torque/power and longer motor operation before reaching temperature limits.
Analyzing the Test Data
Additional analysis of the data found in Nategh’s dissertation was carried out at LORD Corporation. Hotspot temperatures from the dissertation were tabulated based on the potting materials thermal conductivity (varnish only, 0.25 W/m•K; Epoxylite, 0.9 W/m•K; and CoolTherm SC-320 encapsulant, 3.2 W/m•K), applied current and coolant flow rate. Data were analyzed as a three-variable general factorial experimental design using common statistical analysis software. The results demonstrated that end winding temperatures relied strongly on the current and thermal conductivity of the impregnation material, but there was no statistically noteworthy dependence on coolant flow rate.
Statistical analysis further demonstrated that with the CoolTherm SC-320 encapsulant, it is possible for the average end-winding temperature to be 25 °C to 45 °C lower than the varnish-only motor; and 10 °C to 15 °C lower than the epoxy-impregnated motor (Figure 1). Compared to just varnish alone, the expected achievable current needed for reaching the maximum temperature of 150 °C is 14% higher with Epoxylite and 26% higher with CoolTherm SC-320 encapsulant.
Since the current is directly related to the torque, enhanced thermal performance of the potted motors offers a number benefits:
- Higher Power Density – More horsepower/torque from a similarly-sized motor; the same horsepower/torque from a smaller motor; and the ability to remove copper or steel from the motor.
- Better Reliability – Low-temperature operation prevents rapid degradation of insulation, increasing the lifetime of motor insulation.
- Lower Copper Losses – Lower operation temperatures translates to lower copper losses; less resistance to current flow = lower I2 R losses.
Independent Potting Study
To separately verify the effectiveness of using high conductivity thermal materials to advance motor performance, LORD Corporation worked with Advanced MotorTech, an engineering consulting and services company. Advanced MotorTech is involved in the research, fabrication, testing, development and re-design of high-performance and industrial machines.
Advanced MotorTech tested the potting compound on a different type of motor from the ones employed in the LORD Corporation research. They purchased Class F, 3 hp, 182T frame, completely enclosed fan-cooled (TEFC) motors with a rated rpm of 1760; voltage: 208-230/460, three-phase, 60 Hz; full-load current: 8.4-7.8/3.0. For the test procedures, they were aware that using a resin in a standard motor could advance the performance of a motor by making the motor run cooler or making it run harder without going beyond its temperature rating. Advance MotorTech went into the testing with no preconceived notions of the outcome.
Six thermocouples were installed on each motor end (fan-end and drive-end), two per winding base, for a total of 12 thermocouples measuring winding temperature.
Thermocouples were also added to the motor skin in order to monitor the outside temperature. A “quick, full-load test” was used for testing four “as-received” motors. From that testing, two motors with similar power, current and temperature rise were selected for subsequent tests.
A controlled test for equal comparison was carried out on the unpotted motors. The motors were attached to a DC generator. A digital meter provided readout of the torque transducer and a dynamometer precisely controlled the power output of the generator. To determine an accurate comparison between the motors, the testing was performed in the same room, with the same load, meters and generator; and the same ambient temperature (24.8 °C). During the testing, the motors were made to run until they were thermally stable. Temperatures increased in the motors over time. The shaft-end ran warmer and the fan-end ran cooler, and both motors functioned according to the manufacturer’s instructions.
Of the two motors chosen for the testing, one was encapsulated with CoolTherm SC-320 silicone encapsulant and the other was left unpotted. CoolTherm SC-320 resin was mixed with CoolTherm SC-320 hardener for the potted motor testing. In this work, the CoolTherm SC-320 silicone encapsulant was poured into the motor, even though the encapsulant can be applied with automatic meter/mix/dispense equipment or handheld cartridges. A vacuum was used for minimizing air bubbles. The encapsulant was allowed to cure for 24 hours at room temperature.
It can also be cured in a faster manner at 125 °C for 60 minutes. The insert was removed and the air gap cleaned after the curing process. During the encapsulation process, workers at Advanced MotorTech discovered that CoolTherm SC-320 encapsulant displayed none of the “strong” odor or “eye-irritating” fumes commonly noticed in other epoxy compounds.
The same load conditions were used for the unpotted vs potted motors – a full-load test (100% torque). Testing results presented that the average overall temperature was 7 °C cooler with the potted motor (54 °C compared to 61 °C for the unpotted control motor). The temperature difference between the fan and drive sides was just 3 °C compared to 5 °C to 6 °C for the unpotted motor. Thus, the potted motor, encapsulated with CoolTherm SC-320 encapsulant, ran cooler than the unpotted motor. (Figures 1 and 2).
.jpg)
Figure 1. Average Coil Temperature – Drive-End.
.jpg)
Figure 2. Average Coil Temperature – Fan-End.
Both motors were also tested at overload conditions (164% and 174% rated torque) in order to determine the thermal effects under extreme conditions. The potted motor averaged 30 °C cooler than the unpotted motor at the highest torque. This is a major difference and could lead to a projected eight-time longer dielectric life. The temperature difference between the fan and drive sides was less in the potted motor (ΔT = 9 °C compared to 16 °C for potted compared to unpotted motors, respectively).
The potted compared to the unpotted motors also showed a dramatic difference in skin temperatures. Testing showed that, while the average skin temperatures were about the same, the potted motor had just an 8 °C difference between the coldest and hottest spots, whereas the unpotted motor had a 20 °C difference. Thus, there was a more even distribution of skin temperature in the potted motor. This can be attributed to the air-gap-filling properties of the silicone resin. CoolTherm SC-320 encapsulant smoothly and evenly fills in all air gaps around the end windings. This results in even differential temperatures around the motor’s frame and enables mitigating any performance and stress issues that might be produced as the motor operates.
Conclusion
The results of testing both at KTH and at LORD Corporation clearly show the benefits of potting with thermally conductive materials. Motors potted with CoolTherm SC-320 encapsulant offer more horsepower and torque at a given temperature and should provide lower copper losses and higher reliability at the same torque (Figure 3). The tests demonstrated an improvement in copper losses of about 10% and a 30 °C difference at the highest applied load and torque. Alternatively, at the same highest temperature, there was a 16% improvement in torque with the potted motor.
.jpg)
Figure 3. Benefits of Potting with Thermally Conductive Materials. (CoolTherm SC-320 encapsulant)
Developed to provide excellent thermal conductivity for electronic/electrical encapsulating, CoolTherm SC-320 encapsulant also retains the desirable properties associated with silicones. The low-viscosity encapsulant provides improved flow-ability while reducing the risk of air entrapment. It has been designed with lower durometer in order to reduce mechanical stress on electronic components and decrease the probability of failure. Its low coefficient of thermal expansion results in lower stresses because of changes in temperature.
Possible applications for CoolTherm SC-320 encapsulant include those in which higher power at lighter weight is required, such as aerospace motors and actuators; motors for electric vehicles; and portable power generation equipment. Better thermal management in electric motors can decrease the current, and thus, the energy needed for providing the necessary power; and can also prolong a motor’s lifetime. Cost savings may also be possible because of the reduced amount of copper wire needed for the motor windings. Thus, savings in energy, weight, and/or cost may all be possible based on the application.
LORD Corporation is continuing to work together with development partners in order to verify the benefits of using high thermal conductivity materials in motor potting applications. These benefits include: higher power densities - reduced steel and copper weight through thermal potting material; advanced efficiency; and enhanced reliability – considerably lower operating temperatures and elimination of hot spots.
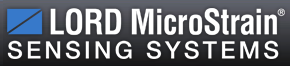
This information has been sourced, reviewed and adapted from materials provided by MicroStrain, Inc.
For more information on this source, please visit MicroStrain, Inc.